The research group consisting of Assistant Professor Motoshi Kaya, Professor Hideo Higuchi, and graduate student Yongtae Hwang (current affiliation: Ariel) of the School of Science at the University of Tokyo discovered that cardiac myosin is responsible for stable and efficient cardiac contraction. They identified molecular properties of cardiac myosin by comparing it with skeletal muscle myosin, as both share a similar amino acid composition. By constructing a simulation model, they found that the molecular properties of cardiac myosin suppress ATP consumption to achieve stable and efficient cardiac contraction. Mutations in cardiac myosin are known to be the leading cause of hypertrophic cardiomyopathy, which can lead to sudden death. The findings of this study are expected to contribute to the clarification of the onset mechanism of this disease. These findings were published in the Proceedings of the National Academy of Sciences of the United States of America (PNAS).
The contraction of the heart occurs through the interaction of actin myofilaments with myofilaments composed of cardiac myosin molecules (cardiac β-myosin). These two types of myofilaments are lined up in parallel with each other in heart tissue. Contraction of the cardiac muscle is generated through the movement of the actin filaments, which is caused by structural changes in cardiac myosin mediated by ATP hydrolysis (power stroke).
Because 80-90% of amino acid residues are shared between cardiac myosin and skeletal muscle myosin, their molecular properties have been considered to be similar conventionally. However, their functions are distinctively different; the myocardium requires the ability to repeat stable contraction and rapid relaxation processes, whereas fast skeletal muscles have a wide range of abilities, generating from large to small forces and from slow to quick contractions.
The research group had previously studied fast muscle myosin, which is a type of skeletal muscle myosin, revealing its molecular properties. To expand on this, they aimed to identify the properties and functions of myosin in the heart by comparing cardiac myosin with fast muscle myosin.
First, to investigate the force-generating properties of cardiac myosin and fast muscle myosin molecules, they isolated cardiac myosin from the left ventricle of the porcine heart and fast muscle myosin from rabbit fast muscle. They artificially synthesized myofilaments consisting of approximately 15 cardiac or fast muscle myosin molecules, respectively. Then, they fixed the myofilaments with resin beads using optical tweezers and measured the force generated upon the interaction of the myofilaments with actin filaments. That force was calculated on the basis of the displacement of the resin beads, as recorded under a uniquely developed single-molecule laser trap microscope. The results showed that these two sources of myosin had completely different waveforms; in particular, cardiac myosin exerted approximately twice as much force as fast muscle myosin. Furthermore, in cardiac myosin, the force often decreased in the direction opposite to the contraction direction. This phenomenon was not observed in fast muscle myosin.
Then, they isolated individual molecules of cardiac myosin and fast muscle myosin and similarly applied the pulling load to the molecules upon their interaction with actin filaments to evaluate their structural changes.
The results showed that fast muscle myosin underwent a structural change, causing the actin filaments to slide in the direction of contraction without yielding to the load (power stroke), and its distribution was concentrated in one structural state. By contrast, cardiac myosin was distributed in three structural states, and it was shown to repeat the transitions between these structural states via a power stroke and a structural change in the opposite direction (reverse stroke).
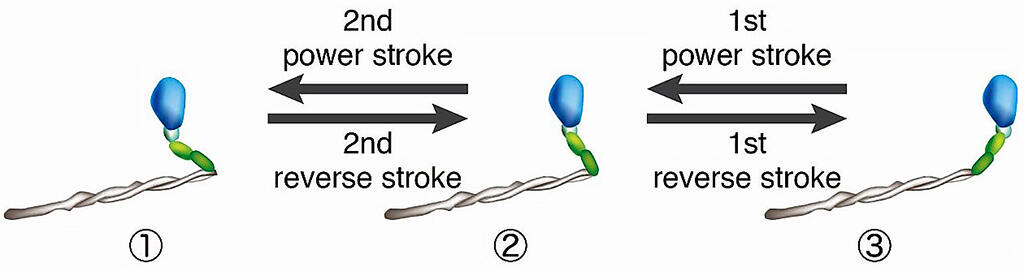
Credit: The University of Tokyo
Next, the research group constructed a simulation model to examine how the reverse stroke characteristic of cardiac myosin affects force generation in cardiac myosin molecules and cardiac contraction. On the basis of the previous measurements, in the simulation, they compared the model in which the reverse stroke occurs (HRS model) with the model in which the reverse stroke does not easily occur (LRS model).
The results showed that reverse stroke frequently occurred in cardiac myosin depending on the increase in load, which allowed more molecules to bind to the actin filaments, maintaining a large force for a long period of time. They also found that the molecules subsequently caused a series of chain reactions from the reverse stroke to the dissociation of actin, resulting in a sudden release of force. Furthermore, a comparison of the ATP consumption rates in the HRS and LRS models revealed the overwhelmingly smaller number of ATP molecules consumed per second for each myosin molecule in the HRS model with the reverse stroke (approximately 1/6).
These results indicate that cardiac myosin achieves efficient contraction by pumping a lot of blood with strong and stable contractions (due to its characteristic reverse stroke) and then reducing pressure with rapid relaxation, thereby maintaining heart function. Since mutations in cardiac myosin are the main cause of hypertrophic cardiomyopathy, the findings of this study are expected to contribute to the identification of the onset mechanism of the disease and the discovery of drugs for its treatment.
In the future, the research group aims to compare cardiac myosin with skeletal muscle-derived slow muscle myosin, which has properties similar to those of the myocardium, and examine how a slight difference in the amino acid composition of these two types of myosin changes the properties of myosin molecules important for structural changes. As explained by Assistant Professor Kaya, "The reverse stroke had not attracted attention because of its structural change being opposite to the power stroke, which produces a contraction force. But our study has shown that the reverse stroke is important for heart function. We hope that our findings will contribute to the identification of the pathogenic mechanism of cardiomyopathy and the development of therapeutic agents."
This article has been translated by JST with permission from The Science News Ltd.(https://sci-news.co.jp/). Unauthorized reproduction of the article and photographs is prohibited.