The Muon is a type of elementary particle and a major component of cosmic rays, so abundant at a rate of one muon per second passing through a palm of each person. The mass of the muon is approximately one-ninth that of a proton. The muon have a lifetime of 2.2 microseconds and a positive muon decays to emit a positron. The muonium, in which a positive muon and an electron are bound, is known as an exotic atom. A research group including Associate Professor Hiroyuki A. Torii at the Graduate School of Science, University of Tokyo; and Dr. Shoichiro Nishimura and Professor Koichiro Shimomura at the High Energy Accelerator Research Organization (KEK), succeeded in precisely determining the hyperfine structure of muonium atoms. They did this by devising a new method of atomic spectroscopy, named Rabi-oscillation spectroscopy, in which the resonance frequency is determined from the time response of only one frequency. Associate Professor Torii says, "In standard spectroscopy, researchers used time-integrated data to investigate the response from atoms by changing the frequency. This time, we came up with a new idea - an inverse way of thinking, so to say - and realized a new method of determining the resonance frequency by solving an inverse problem. The solution is given by comparing the resonance signal with the theoretical simulation of Rabi oscillations, without converting the resonance signals in the time domain to those in the frequency domain." The group's findings were published in Physical Review A.
In the atomic world, allowed states appear as quantum levels at discrete energies, and the frequency of the electromagnetic wave emitted or absorbed during a jump (or a transition) between levels has a frequency sharply determined in accordance with the energy difference between the levels. The accurate determination of this resonance frequency will enable us to precisely test the laws of physics in the microscopic world.
In standard spectroscopy, an atom or molecule is excited by electromagnetic waves such as lasers or microwaves, and the point where the signal is maximized is regarded as the resonance center. In practice, by changing the frequency of the electromagnetic wave, the resonance frequency at which the curve attains the maximum peak is determined from the symmetrical resonance curve obtained by drawing each signal strength on a graph. However, for precise measurements, it is essential to control the experimental conditions, such as the constant power of the electromagnetic wave. In addition, in order to draw precise resonance curves, data at frequencies with weak signal strength, which correspond to the foot of the mountain in the curve are also indispensable. Hence, this method is not necessarily efficient for precise measurements.
The research group focused on the Rabi oscillations caused by the interaction between atoms and electromagnetic waves, where the signal strength of the transition oscillates with time. If the frequency of the electromagnetic wave coincides or is close to the resonance frequency, the signal oscillations are large and slow, but if the deviation (or the detuning) between the two frequencies is large, the oscillations are small and fast. On the other hand, the power of the electromagnetic waves is also a determining factor. The signal becomes large and fast when the power is high and becomes small and slow when it is low. These combinations differ from the cases where the detuning is altered.
By utilizing this feature, both detuning and power can be obtained simultaneously from the combinations of signal strength and oscillation speed. In other words, as the detuning is known, the resonance frequency can be determined by subtracting it from the frequency of the known electromagnetic wave, regardless of the power. It was also found that by fixing the frequency near the resonance center, the accuracy could be doubled compared with that obtained using standard spectroscopy.
In this research, after validating the principle by theoretical simulations, it was experimentally verified using actual atoms at the Materials and Life Science Experimental Facility (MLF) at the Japan Proton Accelerator Research Complex (J-PARC). Through this it was established as a new spectroscopic method, named Rabi-oscillation spectroscopy. Rabi oscillation is a phenomenon that has been in textbooks for decades, but only a few studies have attempted to inversely determine the resonance frequency from the speed of Rabi oscillation. In standard atomic and molecular spectroscopy, it is often sufficient to erase the time information and make measurements either by integrating the signal or by using the equilibrium state after the oscillations. Especially, in high-precision spectroscopy, it is common practice to make observations by changing the frequency under the same conditions. It was considered important to draw a precise resonance curve with a narrow frequency range by taking time to measure with a moderate or weak power.
However, in the spectroscopy of atoms containing short-lived elementary particles and nuclei produced using accelerator facilities, it is necessary to make quick observations with as much power as possible in order to obtain the maximum signal from the limited observation time and limited number of atoms. While power variation tends to be a problem, Rabi oscillations are remarkably fast and easy to observe, at a few hundred nanoseconds. Rabi-oscillation spectroscopy is an epoch-making technique that enables quick, yet highly accurate and efficient spectroscopy.
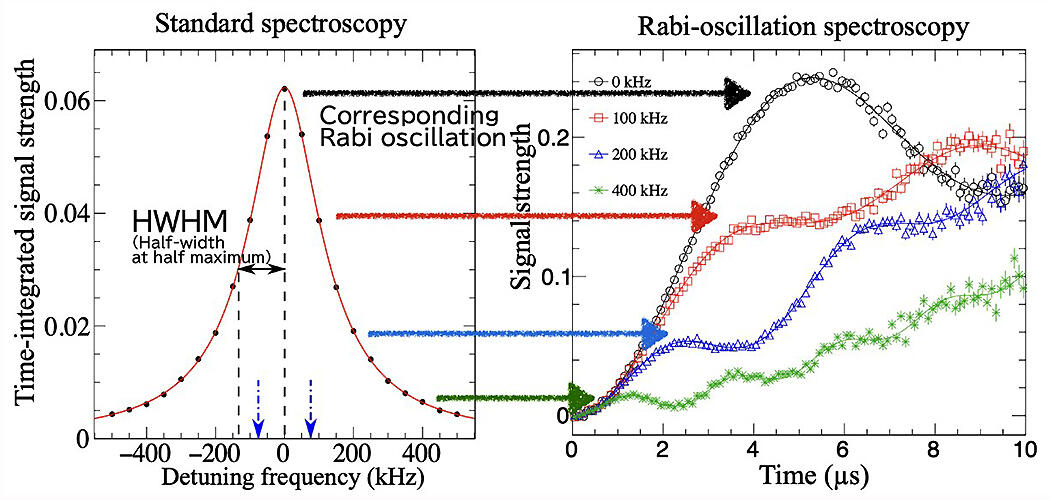
Credit: Hiroyuki A. Torii, Shoichiro Nishimura, Koichiro Shimomura
The research group focused on muonium and has been working on high-precision microwave spectroscopy of its hyperfine structures. A muonium atom is a simple atom in which a positive muon and a negative electron are bound to each other, and is regarded as a light isotope of hydrogen where a proton and an electron are bound. The muon has a lifetime of only 2.2 microseconds, and Rabi oscillation spectroscopy can be effectively applied. Although the microwave was amplified approximately 10,000 times by a resonator, there is a distribution of relative power depending on the location of the atom. The actual analysis required a complicated process where the power distribution and the distribution of atoms were taken into consideration to calculate the Rabi oscillations, and the detuning was obtained so that the measured data could be reproduced. As for the hardware, the excellent time response characteristics and accurate time determination of the silicon strip detector used in the experiment were also important factors for realizing Rabi-oscillation spectroscopy.
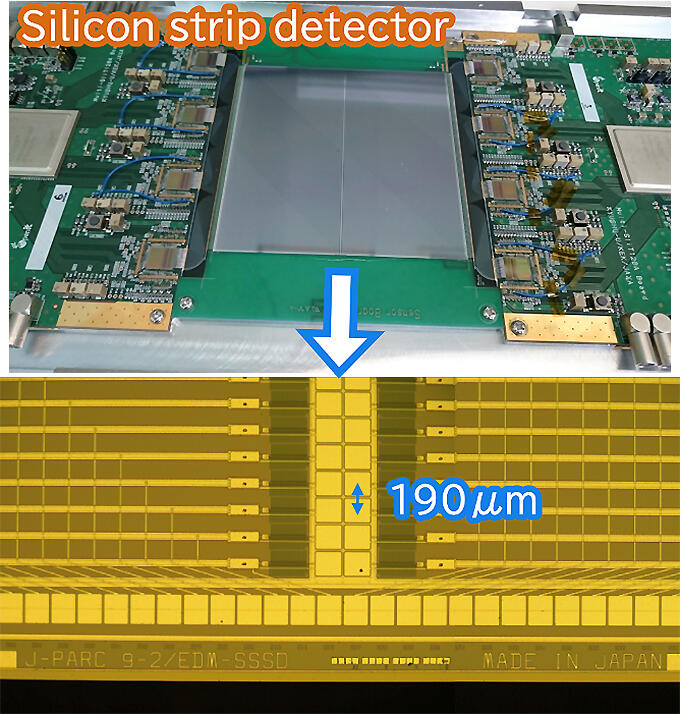
Credit: Hiroyuki A. Torii, Shoichiro Nishimura, Koichiro Shimomura
As a result, the research group succeeded in breaking the record of accuracy in conventional muonium spectroscopy experiments under zero magnetic field conditions. Researcher Nishimura commented, "This time, we conducted the experiment with 1 trillion muons, and we can further improve the accuracy by increasing the number of muons." A high-intensity muon beam line (muon H line) is scheduled to be completed at the MLF later this year. In the future, observing the resonance transition of muonium atoms in a strong magnetic field is expected to achieve an accuracy of two parts per billion, which will surpass the world record by an order of magnitude in a single month's measurement. This will enable determination of the muon mass with a high accuracy and test of the standard model of elementary particle physics, including quantum electrodynamics.
Prof. Shimomura added, "The g-2 experiment in the Fermi National Accelerator Laboratory has revealed a gap between the experimental results and the standard theory predictions for the muon. In the future, we will test the results of the g-2 experiment using Rabi-oscillation spectroscopy on the muon H-line through which a conclusion can be reached within some years."
This article has been translated by JST with permission from The Science News Ltd.(https://sci-news.co.jp/). Unauthorized reproduction of the article and photographs is prohibited.