Driven by the passion to see what is not visible by the naked eye, a variety of microscopic techniques have been developed. Of the existing microscopy devices, electron microscopes have the highest resolution and can see each atom individually. However, observing the magnetic field of each atom was thought to be impossible. A joint development team of Professor Naoya Shibata and Assistant Professor Takehito Seki of the School of Engineering, The University of Tokyo, and Yuji Kohno and other specialists of JEOL has succeeded in the direct observation of atomic magnetic fields, the origin of magnetism, for the first time in the world, using the newly developed Magnetic field-free Atomic-resolution STEM (MARS). It is a revolutionary achievement that will rewrite the history of microscope development. Prof. Shibata commented, "After nearly 10 years of development, we have finally found a lead on the magnetic structure. The magnetic structure changes rapidly in the low temperature range. For example, we would like to work on observing the moment when superconductivity appears." This result was published online in Nature.
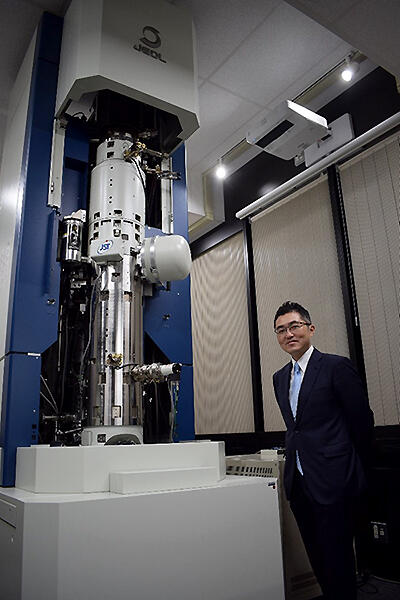
Provided by JST
The performance of current state of the art electron microscopes is 40.5 picometers, achieved in 2017 by a collaborative team of the University of Tokyo and JEOL―this can distinguish objects smaller than the radius of a hydrogen atom (53 picometers). These atomic-level resolutions are achieved by focusing the electron beam with a strong magnetic field lens. Therefore, in a magnetic device, such as a magnetic memory or a spin device, the strong magnetic field of the lens and the magnetism of the sample interact strongly, causing significant change or even destruction of the original structure. If, on the other hand, the magnetic field of the lens is weakened to the extent that the structure is not destroyed, the resolution deteriorates, making it impossible to observe atoms.
In response to this challenge, in 2019, the joint development team demonstrated, for the first time in the world, atomic resolution observations in a magnetic field-free environment by installing objective lenses above and below the sample chamber to completely cancel the magnetic field imposed on the sample. "Until then, there was a big trend for increasing the resolution by aberration correction, so this kind of idea was uncommon, but we thought it was the best way to proceed, and it was realized in cooperation with the fantastic JEOL staff," Shibata said.
Magnetic materials are made up of a combination of different magnetic domains (groups with a constant magnetic field structure), but the magnetic field structure of the domain wall, which is the interface of magnetic domains, is unknown. If this can be understood, the development of high-performance and high-efficiency magnetic materials will be accelerated, and the energy consumption of society as a whole can be reduced. To do so, it is necessary to look at a magnetic field with the resolution of a single atom. Although a magnetic field-free observation environment was achieved, the magnetic field of an atom is so small that the signal cannot be discerned in the presence of the noise in conventional detectors and detection methods.
Therefore, in this study, a newly developed ultrasensitive, high-speed, split-type detector was incorporated into the atomic-resolution magnetic field-free electron microscope MARS (Magnetic-field-free Atomic Resolution STEM), and computer-based image-processing techniques were utilized to observe the magnetic field of iron atoms inside hematite (α-Fe2O3) crystals. For the magnetic field observation, the team used the atomic resolution differential phase contrast (DPC) method, which is a local electromagnetic field measurement technique using scanning transmission electron microscopy (STEM), developed by Prof. Shibata et al.
Hematite crystals have an atomic structure where the Fe atomic layer and the O atomic layer are alternately laminated. As they exhibit antiferromagnetism at room temperature, it is considered that the Fe atoms have a spin structure in which the directions of the magnetic moments are staggered. When the crystals are observed by the conventional STEM method, Fe atoms can be observed; however, of course, it is impossible to capture the information of the magnetic moments.
In the DPC method, the electromagnetic field is visualized by utilizing the phenomenon in which the incident electrons from an electron microscope are deflected by an electric field or a magnetic field, but on its own, this does not allow for the magnetic field to be observed. As there is a strong electric field between the nucleus and the electron cloud inside the atom in addition to the magnetic field, the magnetic field deflection phenomenon is hidden by up to 99% by the overwhelming electric field. To visualize the magnetic field of atoms, a special image processing technique was developed and conducted to cancel the contribution of the electric field by subtracting the signals of the Fe atoms with magnetic moments in the opposite direction in the DPC image, leaving only the signals of the magnetic field. "From the structural information, we succeeded in extracting only the magnetic field by subtracting the influence of electric field between the parts where it is symmetrical," Kono said. When hematite was observed at room temperature and a low temperature of 113 K, a magnetic field was observed along the layers at room temperature, but at low temperature (horizontal), the direction of the magnetic field was rearranged into a longitudinal layer structure (vertical). This is consistent with the simulation results.
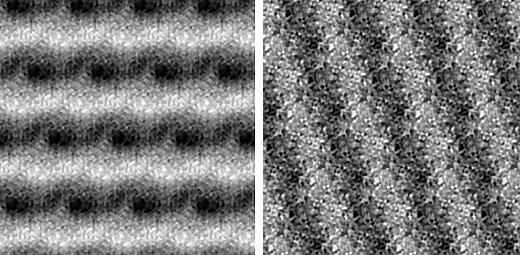
Provided by JST
"This time, in order to confirm whether the magnetic field structure is really visible, we used materials that have a well-predicted magnetic field structure, but next, we would like to make some observations of ferrimagnetic materials and magnetic domain walls," Shibata said. Izumi Oi, President of JEOL, said, "As a Japanese company, we believe it is our mission to be a step ahead of the rest of the world. In this context, we have been able to produce results in MAAS ahead of the world. We wish to develop a variety of applications by working with Japanese universities and industry."
This article has been translated by JST with permission from The Science News Ltd.(https://sci-news.co.jp/). Unauthorized reproduction of the article and photographs is prohibited.