Solid oxide fuel cells (SOFCs) are expected to be a clean energy source because of their low carbon dioxide emissions and high power-generation efficiency. Oxygen-ion conductors, such as yttria-stabilized cubic zirconia (YSZ), are used as solid electrolytes in SOFCs. However, there is a problem in that ionic conductivity drops considerably at interfaces between the numerous crystal grains (grain boundaries) existing inside materials. It has long been proposed that the cause of this drop is the distribution of space-charge layers at the nanometer scale near grain boundaries. It is extremely difficult to observe this layer directly, and the fundamental question of whether space-charge layers really exist at grain boundaries remained unanswered.
Assistant Professor Satoko Toyama, Lecturer Takehito Seki, Project Associate Professor Bin Feng, Project Professor Yuichi Ikuhara, and Director and Professor Naoya Shibata of the Institute of Engineering Innovation, School of Engineering at the University of Tokyo successfully observed the space-charge layer directly inside the solid electrolyte of a fuel cell for the first time, and their findings were published in Nature Communications.
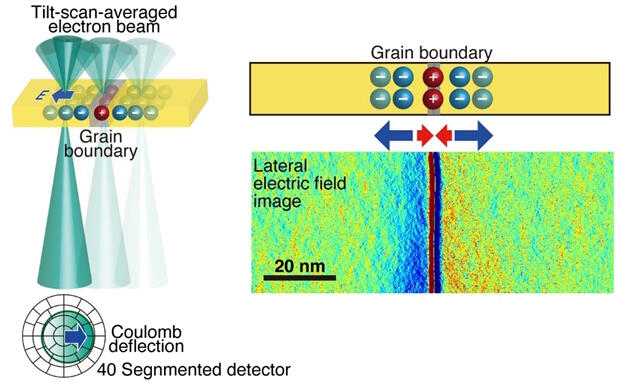
Provided by the University of Tokyo
In recent years, great progress has been made in the electric-field- and charge-observation technique that employs the differential phase contrast (DPC) method with scanning transmission electron microscopy (STEM). In particular, the research group succeeded in locally and quantitatively observing two-dimensional electron gas at semiconductor heterojunctions.
In this observation, the research group employed the tilt-scan averaged DPC (tDPC) method, which they developed to quantitatively observe the electric field and charge at crystal interfaces. The research group performed high-resolution electric field observations on the grain boundaries of YSZ and thereby directly measured the electric field and charge at the nanometer scale, resulting in successful demonstration of the existence of space-charge layers.
The observations were realized using a proprietary system that performs the tDPC method and a magnetic field-free atomic resolution scanning transmission electron microscope (MARS) equipped with an ultrafast, high-sensitivity segmented detector. Furthermore, by performing electric field observation, atomic structure observation, and compositional analysis on precisely controlled YSZ model grain boundaries using the twin-crystal method, the researchers clarified differences in the space-charge layers at each grain boundary and their correlation with the atomic structure.
The atomic-resolution high-angle annular dark field (HAADF)−STEM images of the four observed YSZ grain boundaries and yttrium concentration profiles obtained using STEM energy-dispersive X-ray spectroscopy showed that various crystal orientations lead to different grain boundaries, which in turn yield distinct yttrium concentrations. The horizontal electric field observation image obtained using the tDPC method and its line profile show an electric field emanating from only one of the two grain boundaries with different crystal orientations. Analysis revealed that the center of the grain boundary is positively charged in this emanating electric field and that negative charges exist in the space-charge layer around the center.
Comparison of the distribution of the electric field by location for the four observed grain boundaries in this study showed that the electric field differs substantially for each type of grain boundary and that large space-charge layers exist in the two grain boundaries containing a high yttrium concentration. Furthermore, the space-charge layer is small at grain boundaries where yttrium is not highly concentrated. Negative charges in the space-charge layer around grain boundaries were attributed to be caused by oxygen vacancies, which act as ion-conducting carriers, repelling positive charges in the center of grain boundaries, causing depletion. Conversely, as yttrium substituted at zirconium sites is negatively charged, it is attracted to the positive charge in the center of the grain boundary, resulting in its high concentration at the grain boundary. It is presumed that the lack of conductive carriers at grain boundaries where oxygen vacancies are depleted leads to a decrease in oxygen-ion conductivity.
This research is the first to succeed in quantitatively observing space-charge layers at individual YSZ grain boundaries. Furthermore, the amount of charge in the space-charge layer varies greatly depending on the grain boundary type and is correlated to the grain boundary crystal orientation, atomic structure, and yttrium segregation at the grain boundary.
Grain boundaries with no space-charge layers at all are also observed, and the preferential formation of such grain boundaries in materials is expected to lead to a dramatic improvement in the performance of ionic conductors. Space-charge layers at grain boundaries are thought to have a significant effect on conduction properties not only in YSZ, but also in other ionic conductors, such as those used in lithium-ion batteries.
Until now, there has not been a measurement technique that enables the direct observation of space-charge layers, and the cause of resistance to ionic conduction in these materials has not been identified. The emergence of the new observation technique can provide a crucial breakthrough for understanding the mechanisms underlying the properties of various battery materials.
Journal Information
Publication: Nature Communications
Title: Direct observation of space-charge-induced electric fields at oxide grain boundaries
DOI: 10.1038/s41467-024-53014-w
This article has been translated by JST with permission from The Science News Ltd. (https://sci-news.co.jp/). Unauthorized reproduction of the article and photographs is prohibited.