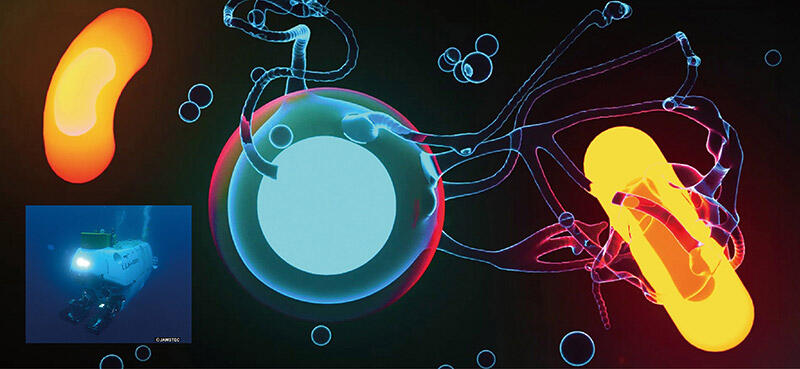
The simple question of where we come from remains an unsolved mystery in 2020. How did any life form, including humans, come to be on Earth, how did life evolve, and how has it prospered? It is a mystery that all of us have wondered about. It has also intrigued and tormented many scientists. A research group made up of members from the Japan Agency for Marine-Earth Science and Technology (JAMSTEC) and the National Institute of Advanced Industrial Science and Technology (AIST) is currently trying to unlock the mystery of life by searching for MK-D1, a new type of ancient organism found in deep-sea sediments.
All living things on Earth belong to one of three classifications: eukaryotes, bacteria and archaea. Of the three, it is eukaryotes that have the nucleus that stores the genome (the blueprint for life) and mitochondria cell organelles that generate energy. Bacteria and archaea, on the other hand, do not have cell organelles and are known as prokaryotes. These are considered to be the ancestors of eukaryotes, which include humans. Meanwhile, microbes, a word often found in school textbooks, is used as a catch-all for all organisms that are invisible to the naked eye, therefore they include prokaryotes and some eukaryotes.
Bacteria and archaea--From the names alone, you might have the impression that the latter would be something that has lived on Earth since ancient times, an organism utterly removed from humans. In actual fact, we may be more closely linked to archaea than you would expect. In recent years, analysis of the content of their DNA reveals that archaea have more in common with human beings than bacteria. From this discovery, an important process of biological evolution has emerged. It is the scenario in which all living things started out either as bacteria or archaea, and then from archaea, eukaryotes were born.
Family tree of all living things
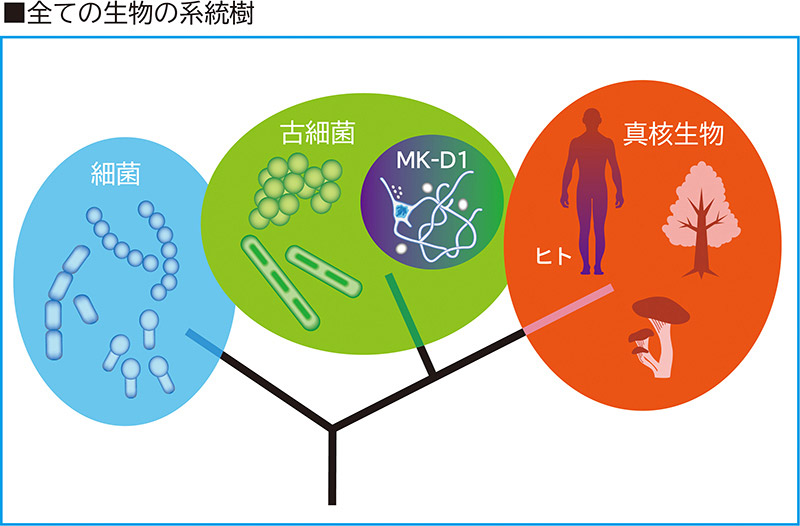
The key to the mystery lies deep in the ocean
So, how did archaea evolve into eukaryotes? While the DNA may be comparatively similar, there are significant differences in the appearance of archaea and eukaryotes, as well as in their life-sustaining mechanisms. What on earth happened during the change from archaeon to eukaryote, the human ancestor?
The key to unlocking this mystery lies in the depths off the south coast of Shikoku, known as the Nankai Trench. At depths of around 2500m are methane vents producing gas thought to have been abundant in ancient times. Due to the extreme lack of oxygen, this is thought to be an environment very similar to that existing at the beginning of the evolution of life.
Senior researcher at JAMSTEC, Dr. Hiroyuki Imachi, focused on sediments taken from the seabed here. Internal investigations revealed a large volume of archaea never before seen. Yet, regarding the archaea at that time, "all we knew was that it was a new previously unknown type, and its classification."
But, says Dr. Imachi, reflecting on the start of his research in 2006, "because there were a lot of that particular archaeon in the sediment, we imagined that the archaea played an important role in the sediment. We believed that if we were able to culture it, we would make some biological and ecological discoveries." It was the start of continuous effort to probe the identity of the new archaeon discovered deep under the sea.
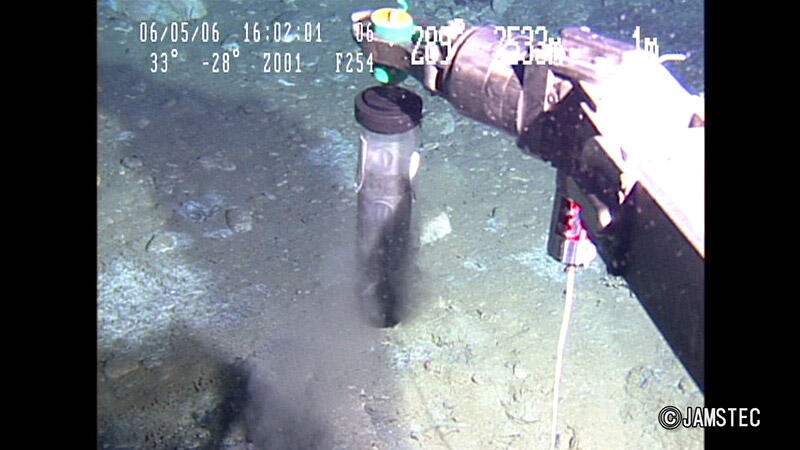
Culturing the mystery archaea--a 10-year mission
Culturing is an artificial technique for growing and propagating specific organisms. For example, if you want to investigate the behavior of a microbe, essentially you need to increase the number of organisms of that type up to a certain quantity. Therefore, if different organisms start multiplying or if the organisms you are targeting start to die, then you have to start the experiment all over again. Here, the target was an unknown archaeon, so Dr. Imachi's research began with developing special equipment to culture the microbe.
It was five years on from the start of the research before the target archaea were first extracted from the sediment. However, there was a big problem. When Dr. Imachi moved the target archaea to a different container in order to increase them, different microbes multiplied and the archaea suddenly all died.
Dr. Imachi's team needed to succeed with the culturing, so they made tweaks to the process. In order to prevent the multiplication of microbes other than the target archaea, they added more varieties of antibiotics. They also started to use quantitative PCR testing to measure the amount of archaea on a monthly basis. Normally, it is easy to see the proliferation of microbes in fluid because it becomes progressively cloudier. However, the growth of the newly-discovered archaea was incredibly slow so even if they grew to the maximum level, the fluid remained as transparent as tap water.
"In microbiology, the maxim is that you can see if bacteria are growing as soon as you look. We did not expect the changes to be at a level invisible to the human eye, and it was a significant observation," says Dr. Imachi. From this alone, "We felt that we were winning if our monthly data showed the archaea increasing."
Archaeon MK-D1 has tentacles
It took 12 years of culturing the new archaeon before it was named MK-D1, but it had a totally different look from any other archaeon found to date. Observing its form through a powerful microscope, it has legs coming out of it like tentacles. Meanwhile, like many other archaea, it had no nucleus inside it and its structure was relatively simple. The next goal for the research group is going to be finding out how MK-D1 lives--in other words, how it produces the energy needed to survive--and the mechanism by which it does so.
The clue to solving this new mystery lies in the "genome" briefly mentioned at the start of this article. Originally, "genome" is the word for all of the genetic information held by a living organism. Of that, the parts required to build the actual body of the organism are called "genes." In actual living things, there are multiple chemical reactions to generate energy, and in most cases, they are interlinked. As such, to find out how each of these reactions work, you need to investigate not only each gene but all of the genetic information--the genome.
AIST researcher Dr. Masaru K. Nobu has been a very helpful contributor on this point. Having studied engineering at university, it was Dr. Nobu who realized that they were looking at an MK-D1 genome, because of his awareness that data would not just have to be analyzed: they also needed to predict the progress of the chemical reactions.
"Many prokaryotes have a self-contained reaction path so they can generate energy within their own cells. But this new MK-D1 only has fragments of the reaction paths to make energy. So, we theorized that it has a completely different strategy for obtaining energy that has not yet been discovered."
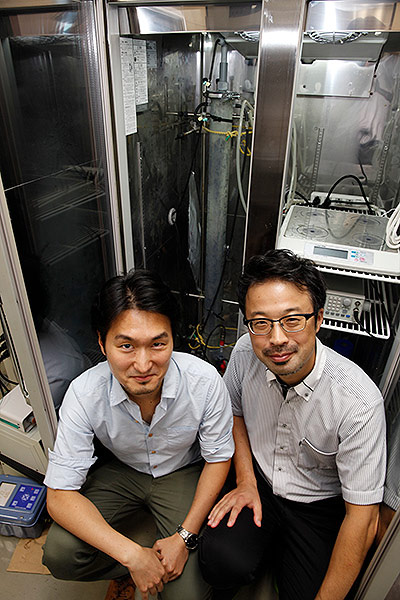
Survival strategy of an "incomplete" organism
So, what could the "completely different strategy for obtaining energy" that Nobu mentioned be? Many microbes, including archaea, build themselves by breaking down externally sourced nutrients to obtain energy, then finally dividing to increase their number. The MK-D1 on the other hand cannot synthesize its own energy. In other words, it is unable to build itself or reproduce itself. To borrow a phrase from Dr. Imachi, then, how does MK-D1, "the least complete living thing ever found," survive?
Actually, in the trialing of different culturing methods, they found that MK-D1 would often turn up around different kinds of microbe. It was also found that the surrounding microbes needed the hydrogen produced when MK-D1 synthesized energy. Moreover, archaea able to survive in oxygen-poor environments like the MK-D1 are unable to produce energy smoothly when there is too much hydrogen present. Here is where the MK-D1 survival strategy emerges. In return for supplying hydrogen to the surrounding microbes, those microbes synthesize some of its energy for it.
Furthermore, as the key to this symbiotic relationship, Dr. Imachi and Dr. Nobu focused on the "tentacle-like protrusions." The current hypothesis of the research group is that the MK-D1's tentacles entangle surrounding microbes, at which point a mutual transfer of materials takes place.
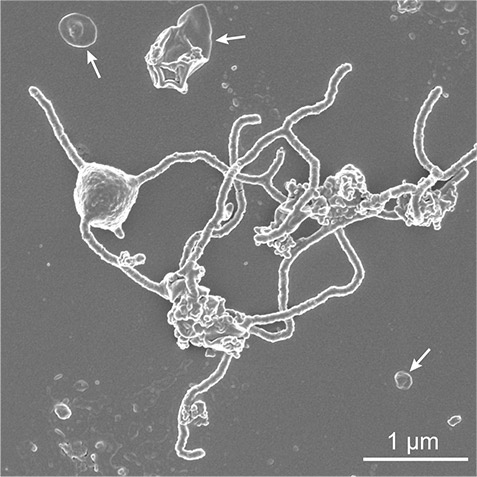
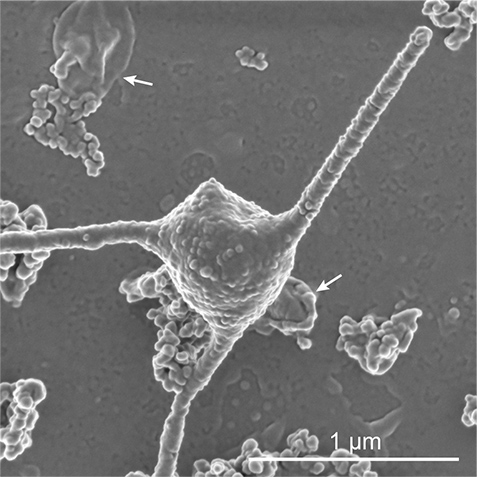
Diagram of the evolution of life, incorporating the discovery of MK-D1
Further analysis of the genome revealed several genes previously thought to be unique to eukaryotes. These discoveries mean that MK-D1 is "the archaeon closest to a eukaryote". Nobu makes the following projection of the history of evolution of our ancestors, based on these discoveries related to MK-D1.
2.7 billion years ago, a dramatic rise in oxygen levels began on the planet. This was a tremendous change that threatened the survival of organisms that live in an oxygen-free environment. At this time, those organisms that chose to live in oxygen-free environments began to be joined by those synthesizing energy more efficiently through the use of oxygen.
The archaea that were our ancestors chose the path of survival in a new oxygenated world, together with microbes that could deal with oxygen. MK-D1, once it had built symbiosis with surrounding microbes, eventually united with them over a long period of time to give birth to the first eukaryote. Later, this microbe probably changed into mitochondria, the cell organelles dedicated to energy production.
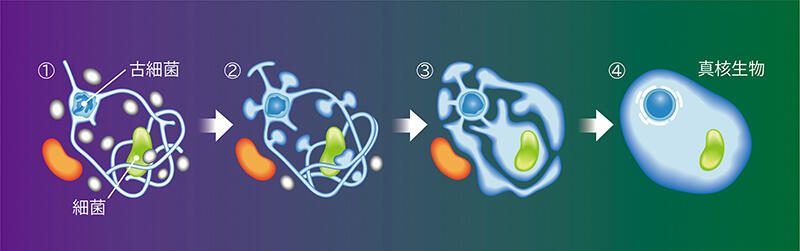
Next, the research group will continue to study the MK-D1 in order to shed further light on the evolutionary process. Dr. Imachi is enthusiastic. "For example, we do not yet know whether the tentacles of the MK-D1 are in fact able to entangle other microbes. In order to find out what they actually do, we are investigating what the tentacles are made of. It would also be interesting to look at whether they can actually incorporate the microbes when we are culturing it while varying the oxygen level."
Research into the origins of life serves to remind us of the string of happy coincidences that led to the "miracle" of our existence. I hope we can continue to stay abreast of the quest to unlock the secrets of this miracle through scientific eyes.
Dr. Hiroyuki Imachi, Senior Researcher, JAMSTEC
Graduated from the Department of Civil Engineering and Architecture, National Institute of Technology, Tokuyama College in 1996. Completed Ph.D. at the Nagaoka University of Technology in 2003. After working as an assistant and a researcher, he has been a senior researcher at JAMSTEC since 2009. His research field is microbial ecology. In order to understand the life of anaerobic microorganisms, Dr. Imachi is currently undertaking research cultivating viable but non-culturable microorganisms, known as "microbial dark matter."
Dr. Masaru K. Nobu, Researcher, AIST
Graduated from Carleton College, USA in 2011. Completed his MSc at the University of Illinois in 2013, and then his PhD in 2017. Reached current post in 2017. Research interests are microbiology and genome science. From genomes and genes, which are both the blueprint for life and record of the history of life, he is aiming to reveal the evolutionary path taken by life over the past 4 billion years and to uncover and harness the hidden power of microbes.
Original article was provided by the Science Portal and has been translated by Science Japan.