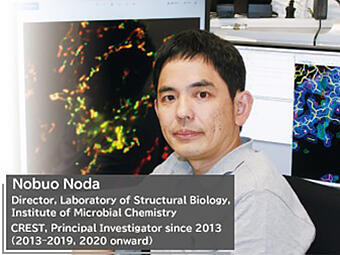
Collaborative research on different fields Devotion to clarification of the phenomenon
Autophagy is a process that recycles, not breaks down, proteins in the body. The substance to be degraded is encapsulated in a lipid membrane structure called an "autophagosome" and is then degraded (Fig. 1). In other words, the autophagosome sorts substances to be degraded from those that are not. Dr. Nobuo Noda, Director of the Laboratory of Structural Biology, Institute of Microbial Chemistry, is currently studying the mechanism underlying autophagosome formation. Dr. Noda is also the principal investigator for the project "Autophagy dynamics driven by multi-layered higher-order structural components" in the research area of CREST "Spatio-temporal dynamics of intracellular components."
Dr. Noda first learnt about the autophagy phenomenon in his postdoctoral work. At graduate school, he specialized in X-ray crystal structure analysis, which helps examine protein crystal structures using X-ray irradiations. After obtaining his doctoral degree, he joined Professor Fuyuhiko Inagaki's laboratory at the Graduate School of Pharmaceutical Sciences, Hokkaido University (at the time) as a postdoctoral researcher. Prof. Inagaki was conducting protein structural analysis using nuclear magnetic resonance (NMR) without crystal formation.
Reminiscing on those days, Dr. Noda said, "I wanted to approach the phenomena of life via structural analysis of proteins. However, the crystal structure of a protein may differ from its actual structure inside the body or during a reaction. I felt that there was a significant gap in knowledge on this phenomenon. I wondered whether it was a good idea to simply study these crystal structures. I wanted to devise a method to observe a dynamic state that is closer to the actual phenomenon." During the period when Dr. Noda was inspired by protein structural analysis in the solution state using NMR, Yoshinori Ohsumi, a specially appointed professor at Tokyo Institute of Technology, began collaborating with Prof. Inagaki's laboratory.
Dr. Ohsumi, who discovered several Atg genes related to autophagy, requested Dr. Inagaki for joint research to elucidate the function of the genes, and Dr. Noda decided to investigate the structure of the Atg protein produced by the Atg gene. "It is embarrassing, but I didn't even know the term "autophagy" until I started working on that collaboration. I hurriedly tried to learn about it as much as I could, and soon realized that it is a really interesting phenomenon. I fell in love with it all at once." At that time, although it was known that autophagy is involved in organelle and protein decomposition and regeneration, its underlying mechanism had barely been clarified. He felt that genes involved in autophagy was a worthwhile area of research.
A lipid transport protein with a huge hydrophobic cavity inside
From this, Dr. Noda's autophagy research began. Proteins, which are synthesized as chains of amino acids, adopt unique conformations defined by their sequence, which in turn defines their functions. To clarify the structure and function of the Atg protein, which was poorly understood at that time, Dr. Noda produced Atg protein crystals and attempted to analyze their structure. Several Atg proteins have regions with specific three-dimensional structures. In addition, these proteins have regions called "intrinsically disordered regions" that show fluctuating structure. Hence, crystallization of the proteins was difficult. Dr. Noda succeeded in forming the crystals by discarding these intrinsically disordered regions and then began to determine their structures.
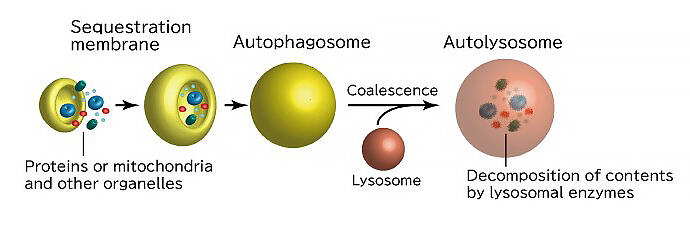
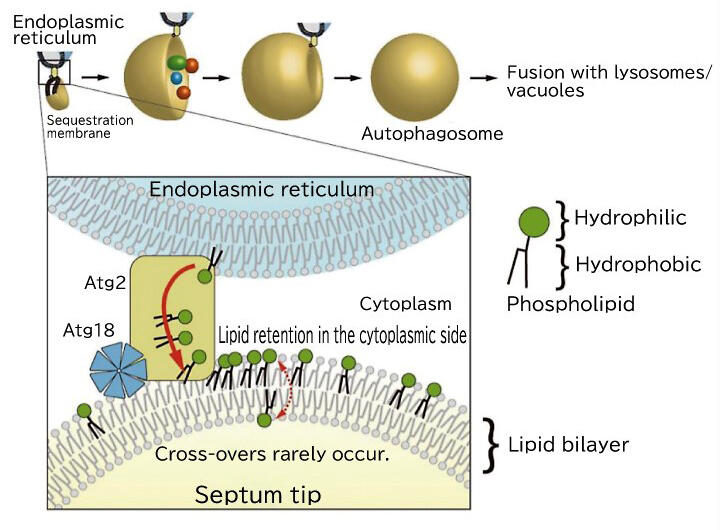
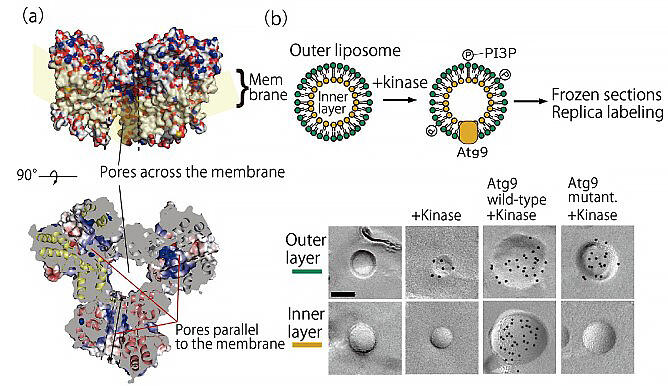
The remaining challenges were Atg2, the largest of the major Atg proteins, and Atg9, the only membrane protein among the Atg proteins. Atg2 was determined to form a complex with the lipid-binding protein Atg18 and combine with the sequestration membrane, the precursor of autophagosomes. In 2017, electron microscopic analysis revealed that it was as long as 20 nm (nano as 1 billion-fold) in the form of a rod. After Dr. Noda succeeded in partial crystallization of Atg, he analyzed the structure in 2019 and confirmed that it had a huge central cavity.
Because the cavity was composed of mostly hydrophobic amino acids, Dr. Noda hypothesized that it would bind lipids. He attempted to crystallize the complex with phospholipids, which was a brilliant idea. Ultimately, he succeeded in crystallizing the complex with phospholipids incorporated into the cavity. This structure revealed that Atg2 is a lipid transport protein that functions in extracting and carrying phospholipids required for producing autophagosomes from the endoplasmic reticulum (Fig. 2).
In contrast to this, autophagosomes are sac-like organs composed of lipid double membranes. Even if Atg2 carries the phospholipids in the outer layer on the cytoplasmic side of the double membrane, the double membrane cannot be extended without the lipids in the inner layer. Therefore, Dr. Noda focused on Atg9, a membrane protein whose role was unknown. Although efforts had been made to crystallize Atg9 and investigate its structure, for a long time, its crystals could not be obtained. The breakthrough was made in a joint research with Professor Masahide Kikkawa of University of Tokyo Graduate School of Medicine, who participated in the same CREST project. The three-dimensional structure of Atg9 was verified using cryo-electron microscopy, in which is Dr. Kikkawa specializes. Atg9 was determined to have a hydrophobic cavity similar to that of Atg2, and Dr. Noda thought that it may have a lipid-carrying role.
The function of Atg9 was verified using artificial lipid membranes in vitro, and it was demonstrated that it had the function of carrying lipids between the outer and inner layers. The researchers also confirmed that yeast cannot produce autophagosomes when the amino acids that form the cavities containing lipids are mutated. "We deduced that Atg2 and Atg9 worked together to form autophagosomes. I was very surprised at this completely unexpected mechanism," he said.
The idea of 'reversal' provides turning point: expressing function via phase separation
Dr. Noda has been researching the protein function from the perspective of its structure for many years. However, when he was reviewing the schematics he drew for publication of his paper, he realized that the proteins might function by aggregation through their intrinsically disordered regions.
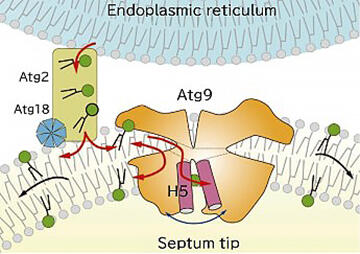
He said, "I was attempting to clarify the function of the protein by its structural analysis; so, for many years, I ignored the intrinsically disordered regions that interfere with it. However, by turning the problem on its head, I realized that the area that I considered an obstacle was important for the protein to function properly." In 2009, a German research group reported on the concept of "liquid-liquid phase separation" in which a specific protein separates and concentrates in a liquid phase that differs from its surroundings. It has since been reported that intrinsically disordered regions are involved in liquid-liquid phase separation.
Dr. Ohsumi also found previously that starved yeast accumulates Atg proteins in one spot before the formation of autophagosomes. Although its function remained unknown, this structure was called a "pre-autophagosome structure (PAS)." Dr. Noda decided to re-evaluate the Atg protein using the liquid-liquid phase separation concept because the PAS is similar to the droplets formed via liquid-liquid phase separation.
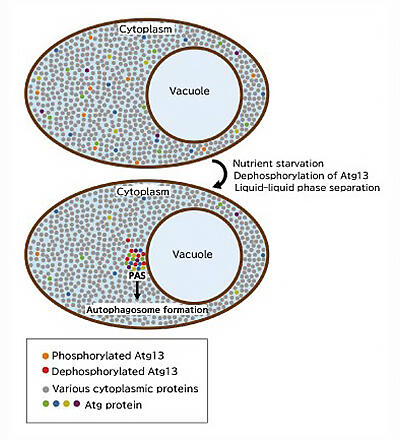
A meeting with fellow researcher at a conference leads to results obtained by maximizing novel analysis methods
First, Dr. Noda decided to investigate the conditions under which Atg proteins work. When observing the state in the cytoplasm, microscopic, not structural, observations are the primary focus. Despite the introduction of fluorescence microscopy, there were no microscope specialists in the laboratory. After days of trial and error, the researchers managed to obtain basic data.
They confirmed that Atg13, which was dispersed by binding phosphate in a nutrient-rich state, dephosphorylates under nutrient-starved conditions and accumulates in the immediate vicinity of the vacuolar membrane to hold the surrounding proteins together and form PAS (Fig. 5). It was determined that the formed PAS accordingly fused to form a sphere, thereby exhibiting the properties of a liquid (Fig. 6a). However, to verify this observation, it was crucial to obtain more accurate dynamics information; hence, Dr. Noda considered joint research with a microscope expert.
He finally met one such expert at a JST-hosted study session, an event where researchers can network with members from other research groups. "Professor Yasushi Okada of Graduate School of Science, University of Tokyo, who is an expert in cutting-edge bioimaging technology, told us that he is interested in autophagy. Since then, joint research with him progressed," he said.
In a collaborative study with Dr. Okada, the group adopted fluorescent microscopy to investigate the movement of Atg13, which exhibits a string-like architecture in the cytoplasm. It was determined that Atg13 exhibits greater motility than that in the cytoplasm even when it is inside the PAS. In other words, it was verified that PAS is a droplet with liquid properties.
To comprehensively observe the Atg protein in the PAS, Dr. Noda also asked Professor Toshio Ando of Nano Life Science Institute, Kanazawa University, who he met at a CREST conference, to conduct a joint research. Dr. Noda was once again blessed with a fortunate interpersonal relationship when he met Dr. Ando, who had developed a high-speed atomic force microscope (AFM) and a technology that helps directly observe the dynamics of proteins. According to him, "Fortunately, Daisuke Noshiro, who was a postdoc at the Ando laboratory, was looking for the next post-doctorate position, so he came to my lab. Hence, high-speed AFM observation was made possible in my laboratory. It is important to have people who can master latest equipment."
When the PAS substances were reconstructed in vitro and observed under a microscope, it was observed that they fused with each other in the same manner as in the cells (Fig. 6a, b). Subsequent observation of the reconstructed PAS using high-speed AFM indicated that the Atg protein moved randomly inside the PAS, while retaining its characteristic structure (Fig. 6c). Dr. Noda utilized the latest analytical techniques in a collaborative study to find that droplets formed by accumulated Atg proteins and liquid-liquid phase separation are positive for PAS, and that liquid-liquid phase separation directly controls autophagy.
Interdisciplinary fusion in the laboratory and a breath of fresh air provided by young colleagues
Mr. Noda's discovery that liquid-liquid phase separation directly controls autophagy is a reminder that liquid-liquid phase separation, which had been studied in a number of fields until then, is involved in various biological phenomena. According to Dr. Noda, "Liquid-liquid phase separation is a very interesting phenomenon. I would like to leverage my knowledge thus far to start research on higher-order life phenomena such as sleep and virus infections." The knowledge on liquid-liquid phase separation increases expectations for the clarifaction of previously unknown phenomena.
The diversity of human resources in the laboratory is an important factor that supports the expansion of Dr. Noda's research. When welcoming new laboratory members, it is recommended that, as much as possible, specialized fields do not overlap, and research progresses in a daily basis in the laboratory through fusion of different fields. "Everyone here is an expert in a different field. I believe that it is rewarding to devote myself to research because I can not only learn new things, but also make use of my experience. I would like young researchers to actively jump into different fields," he encourages. It is not uncommon for research to progress swiftly all at once with the introduction of novel analytical methods and equipment. Young researchers with experience and knowledge cultivated in their respective fields of specialization will bring new life to the laboratory and facilitate great achievements. We all have great expectations for the "borderless" activities of the next generation of autophagy researchers.
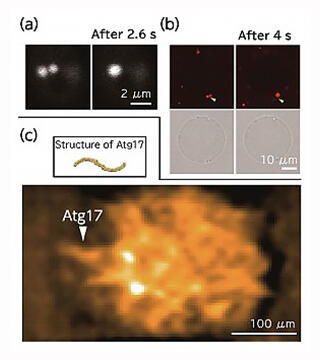