Plants have efficient mechanisms to produce useful substances, including photosynthesis. In recent years, research has been actively conducted to utilize the properties of these plants in industry. Within this field, Keiji Numata, who is a Professor at the Kyoto University Graduate School of Engineering and who leads ERATO "Numata Organellar Reaction Cluster Project", focuses on organelles. By establishing the technology to freely control organelles, his group aims to create innovations in various plant-based industries starting by building basic technology to produce plants with optimal functions for material production.
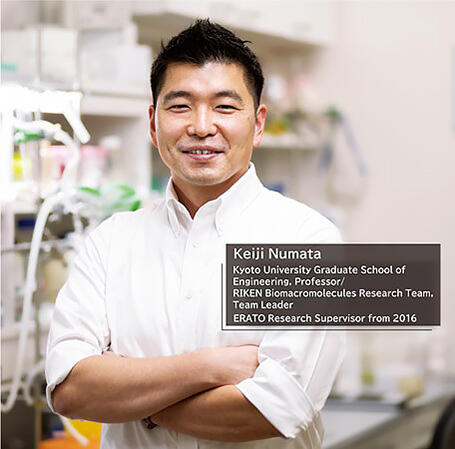
A method easier than genome editing with the goal of producing highly functional new substances
In recent years, the production of substances using living organisms has been attracting attention for the purpose of reducing carbon dioxide and solving energy problems. As typified by photosynthesis, plants can efficiently produce useful substances, and if this mechanism can be utilized not only for food and energy, but also for the production of materials and pharmaceuticals, it is expected to lead to the solution of various social issues. However, the mechanism of substance production in living organisms is extremely complicated, and it has not been possible to completely shed light on the mechanism. However, the mechanism of substance production in living organisms is extremely complicated, and it has not been possible to completely shed light on the mechanism. Therefore, we have not yet reached the stage where we can optimally design organisms to produce the desired substance.
Keiji Numata, Professor at the Kyoto University Graduate School of Engineering and Team Leader of the RIKEN Center for the Sustainable Resource Science Biomacromolecules Research Team, leads the ERATO "Numata Organellar Reaction Cluster Project", and is also one of the people working on research on material production using living organisms. Professor Numata pursued studies in the United States after studying polymer crystal structure analysis in his doctoral course, and he has been involved in the toxicity evaluations of beta sheet structures contained in silk proteins used in regenerative medicine and biomaterials, as well as the development of methods to deliver target molecules to cells. "While focusing on polymer science, I learned how to handle cells and introduce various substances", he stated, looking back at those days.
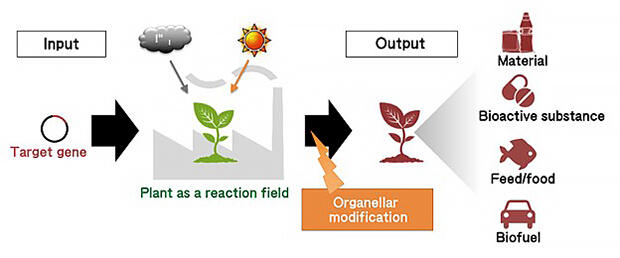
After returning to Japan, Professor Numata pursued research at RIKEN to induce plant cells to produce polyhydroxyalkanoates, which comprise a type of bioplastic. However, even if the nuclear DNA was modified, the expected amount was not obtained. He investigated the reason for this and found that it was due to a lack of substances derived from the mitochondria, one of the organelles. "No matter how much nuclear DNA is manipulated, unless the function of organelles is enhanced, it will be difficult to actually make a large amount of substances necessary for living things, and I thought it was necessary to add an organelle to the target to which the molecule that modifies the gene was sent", he stated.
Since there was no easy-to-use method for modifying organelles and research had not progressed, method development was indispensable. However, DNA modification also required a new method, stated Professor Numata. "Genome editing technologies such as CRISPR-Cas9 have become widely used in the modification of nuclear DNA. However, in the case of plant cells, after editing the nuclear DNA with CRISPR-Cas9, the Cas9 gene needs to be removed from the nuclear DNA. I thought I needed an easier method", he explained.
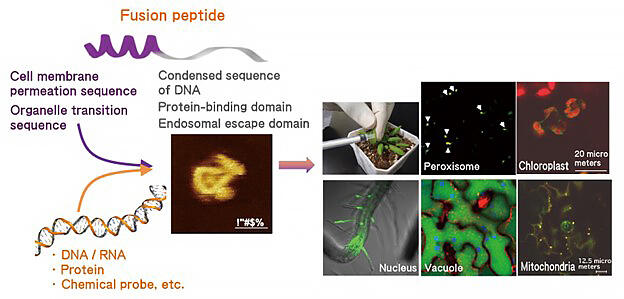
The ultimate goal is to create new materials with high functionality from plants, said Professor Numata. He thought that it would be difficult to achieve that goal simply by establishing individual modification technologies, such as nuclear DNA and organelles, and a new larger goal was set (Fig. 1). Wanting to shed light on all mechanisms related to plant material production based on the functions and interactions of organelles and establish the basic technology for improving plant traits, he launched the ERATO project.
Creation of artificial cell membrane-permeable peptides: DNA introduction into chloroplasts and mitochondria
Initially, in the ERATO project, he organized three groups, the "Fusion Peptide Design Group", "Organellar Modification Group", and "Organellar Interaction Group", and proceeded with research. The Fusion Peptide Design Group is responsible for designing peptides that carry molecules into cells by utilizing their knowledge of polymer chemistry. Using the resulting peptide, the Organellar Modification Group modified the DNA of the nucleus and organelles, and gene expression was to be confirmed by the Organellar Interaction Group using various devices. Currently, considering joint research with other research institutes, studies are being carried out by four groups, including the "Fusion Peptide Utilization Group", in which researchers dealing with algae and human cells participate.
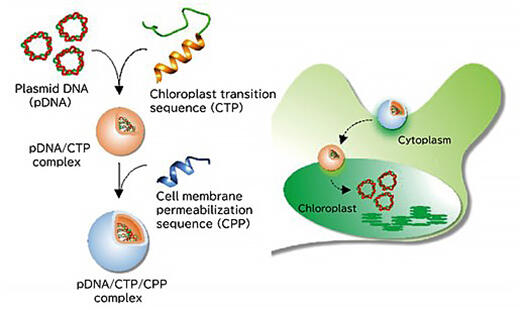
Professor Numata, who had already succeeded in selectively introducing DNA into plant mitochondria, searched for a cell-penetrating peptide (CPP) that more efficiently invades various plant cells (Fig. 2). The group created a library consisting of 55 types of CPPs, centered on peptides commonly used in animal cells, and evaluated their introduction efficiency and introduction mode using plants, such as tobacco, thale cress, tomato, poplar, and rice, as materials. As a result, the introduction efficiency and localization of CPP were found to differ according to the plant species and tissues, and multiple CPPs were identified that are effective against all plant species used.
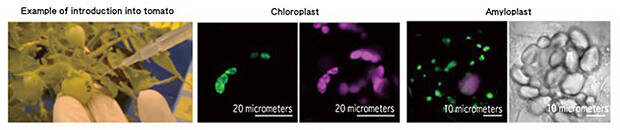
In the confocal laser scanning microscope image on the left, pink indicates the expression of the reporter gene, green indicates chlorophyll, and the bright-field image on the right shows a collection of colorless amyloplasts.
Next, based on the obtained findings, the group attempted to introduce DNA into the plastids of plants and algae. A cluster was created wherein CPP was added to a complex of cyclic plasmid DNA to be sent to the plastid and a peptide that has a chloroplast translocation sequence that promotes uptake into the chloroplast, with positively charged peptides, and it was injected into the leaves of thale cress, a model plant. As a result, the group succeeded in introducing DNA into the chloroplasts as planned (Fig. 3).
Following this success, using a similar technique, attempts were made to introduce DNA into the plastids, chromoplasts, and amyloplasts, which are present in the chloroplasts of tobacco leaves, tomato fruits, and the rhizomes of potatoes, respectively. As a result, although there were differences in the efficiency and introduction time, all experiments were successful, and the expression of the introduced DNA could be confirmed (Fig. 4). According to Professor Numata, this represented the realization of one of the target technologies, but it also had some problems. "The CPP and complex used in this experiment had low introduction efficiency. If we want to produce substances using plant cells, we need to significantly increase the efficiency of introduction". Thus, Professor Numata worked on improving the CPP.
To find an efficient CPP, his group tried all peptides that have been reported to work similarly in the past. However, the ease of permeation varied depending on the target cells. Therefore, Professor Numata succeeded in increasing the stability of peptide-degrading enzymes by adding artificial amino acids that do not exist in nature.
Moreover, depending on how they are taken up by cells, whether or not they are transported to the target organelle will change. For example, for most the endocytosis processes, in which an indentation is formed in the cell membrane and the substance is taken in so as to be surrounded by the indentation, the substance is degraded after being transported to vacuoles in plant cells and to lysosomes in animal cells. With this, it is difficult to reach the target organelle and activate functions such as manipulating DNA therein, even if the efficiency of sending molecules into the cell has been improved.
Success in the direct introduction of a protein
Twenty-times more efficient with artificial peptides
Professor Numata pointed out that these challenges are not unique to plants. In the development of nanocarriers that deliver drugs to the cells of targeted organs, establishing a method to avoid endocytosis is also an issue. "When I was synthesizing various artificial functional peptides aiming at an efficient cell membrane permeability sequence, it turns out that a peptide happens to be in a pathway that is not endocytosis", he said with a smile. As a result of further analysis, it was clarified that macropinocytosis, a phenomenon similar to the uptake mechanism, which had not been reported in plant cells, was induced and the substance was delivered into the cells (Fig. 5).
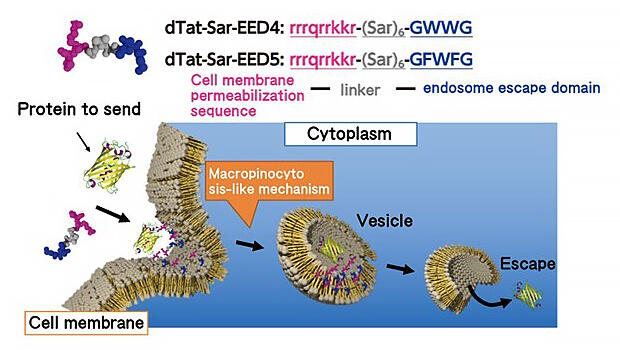
Using the functional peptide (dTat-Sar-EED4) obtained through this, Numata also attempted the direct introduction of functional proteins into plants. His group created a complex of dTat-Sar-EED4 and a hydrolase and tested whether it could be introduced into model plants, such as cultured tobacco cells and Arabidopsis cotyledons (the seed leaf of a plant), and the expression of the enzyme in the cells could be confirmed with a fluorescence microscope (Fig. 6). "It was also confirmed that the introduction efficiency is up to 20 times higher than the CPP used for plant cells so far. It seems to be a strong weapon in modifying organelles in the future", he responded.
However, research on techniques for easily modifying the nuclear DNA of plant cells has also produced results. Professor Numata and others worked on a technology to directly introduce Cas9, a protein that cleaves the genome with CRISPR-Cas9, into cells. When using nano-sized capsules that can contain protein and attaching CPP to this surface and introduction into the callus, which is a mass of undifferentiated cells prepared from thale cress tissue, the group succeeded in efficiently targeting cells and producing typical mutations in the target gene (Fig. 7).
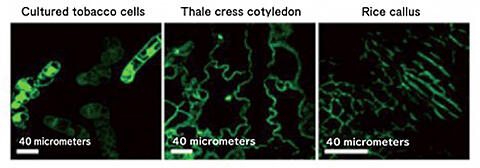
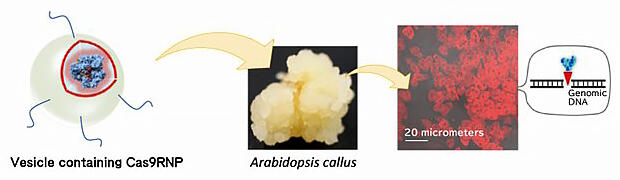
Not only is it easier than the conventional method of incorporating genes required for CRISPR-Cas9 and expressing them inside cells to modify DNA, but there are fewer off-target effects that destroy genes other than the targeted genes. It is expected to be a safer plant genome editing technology. In his latest research, he also succeeded in developing a simpler method wherein nucleic acid is introduced into the plant and the plant is modified by applying a spray (Fig. 8). "These methods will be used to improve the varieties of various practical plants as a versatile and safe genome editing technology", he said.
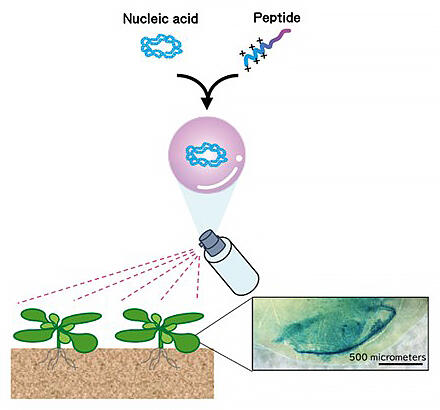
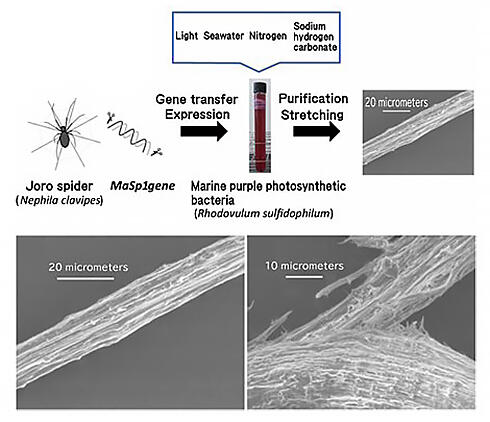
Scanning electron microscope image of fiber obtained from MaSp1 protein (lower left). Scanning electron microscope image of the fracture surface of the fiber (lower right).
Synthesizing spider silk with an eye on industrial applications: Interaction analysis is the next challenge
Applied research using these basic technologies has already begun. One example is research on inducing photosynthetic bacteria to produce spider silk. The silk produced by spiders is lightweight but has toughness comparable to that of steel. Therefore, it is expected to be applied to building structural materials that are required to withstand strong impacts. However, it is difficult to obtain the large amount of spider silk required for use as a building structural material only by extracting it from spiders that are being bred for purpose, and the development of technology for mass production by introducing genes into cells of various organisms is underway.
Professor Numata and others have also succeeded in introducing the gene encoding the MaSp1 protein, which is the main component of Joro spider silk, into purple photosynthetic bacteria. When the obtained MaSp1 was stretched in an organic solvent, the fibers became very similar to spider silk, and when observed with an electron microscope, it was confirmed from the fracture surface that the inside had a fiber structure (Fig. 9). Testing in a large 9 L culture tank is also underway, and if it is put into practical use, it is expected that useful substances can be synthesized while reducing greenhouse gases.
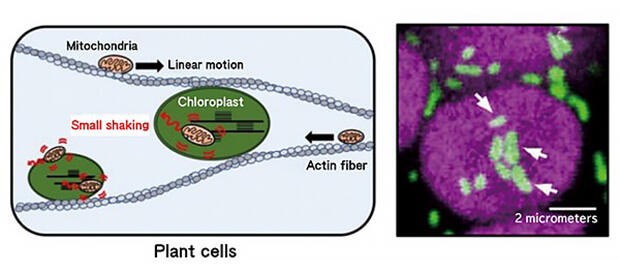
It was clarified that small swaying, which is different from linear motion, correlates with the interaction with chloroplasts.
In addition, research on the synthesis of natural rubber using cultured cells and the production of homogeneous artificial wood has begun. However, further improvements in production efficiency are indispensable for actual industrial applications. "To that end, we will need to clarify the complex interactions between organelles", said Professor Numata as he looked to the next challenge.
As one of the clues, the phenomenon in that mitochondria wiggle due to interactions with chloroplasts has already been reported (Fig. 10) It has been clarified that organelles of different properties interact in the cell and are involved in the metabolism of substances. If these findings are compiled, not only can the function of individual organelles be enhanced, but also the metabolic capacity of cells can be improved by controlling the interaction; this is a solid step towards the future that Professor Numata envisions.