Advances are being made in the development of next generation batteries that are cheaper, have higher performance, and can be used in a wider range of applications, thereby surpassing conventional storage batteries. For use as electrode active materials in their batteries, a subteam led by Professor Kiyoshi Kanamura of the Graduate School of Urban Environmental Sciences at Tokyo Metropolitan University has adopted magnesium (Mg), and another subteam led by Professor Shuji Nakanishi of the Graduate School of Engineering Science at Osaka University has adopted oxygen (O2), and both subteams are aiming to develop a battery with high capacity and high cycle characteristics.
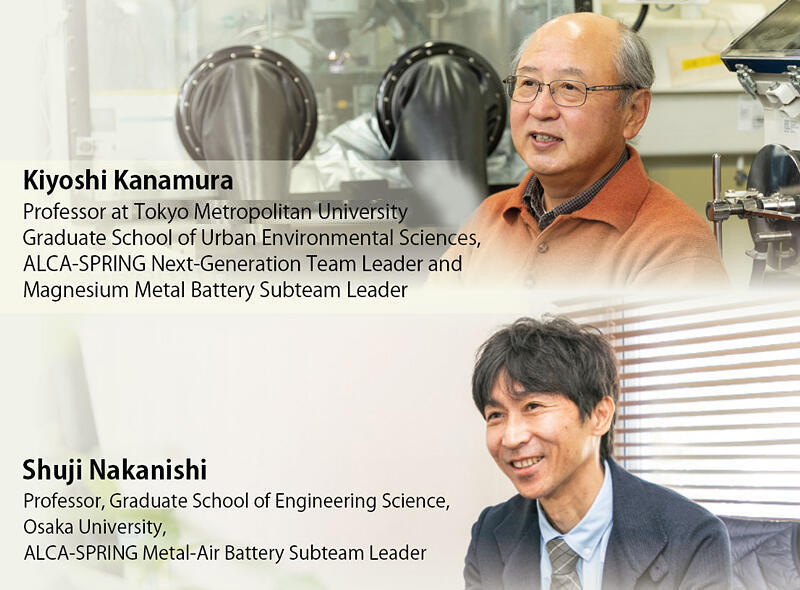
Mg is an abundant resource, but the challenge is to clarify overall picture of a safer battery with higher capacity
Renewable energy is a key technology for realizing a decarbonized society, but it is easily affected by the weather and other factors, so the use of storage batteries in combination with renewable energy is essential to equalize the fluctuating amount of electricity generated from renewable energy. The Next Generation Battery Team, led by Professor Kiyoshi Kanamura of the Graduate School of Urban Environmental Sciences at Tokyo Metropolitan University, is aiming to develop batteries that are even more advanced than next-generation batteries. To achieve this, they are conducting research on storage batteries using magnesium (Mg), which is stable even in the atmosphere and abundant, and on metal-air batteries with the aim of lighter storage batteries.
Kanamura is also the Leader of the Magnesium Metal Battery Subteam. The batteries he is working on use Mg for the anode (the negative electrode in a battery) and oxide or sulfide materials for the cathode (the positive electrode in a battery). Mg is an abundant resource, with 8.5 billion tons in mines alone and an estimated 1,800 trillion tons in seawater. Explaining the potential of magnesium, Kanamura notes, "With a melting point of 650 ℃, Mg is safer than Li and has a higher electrical capacity per volume. Mg also has about six times higher capacity than graphite, which is currently used in lithium-ion batteries (LIBs)."
Although Mg is showing increasing promise as a storage battery material, it also presents many challenges. Mg anodes tends to form an insulating passive film, which makes it difficult for the normal reversible reactions of dissolution and precipitation to occur. This means that optimal charge-discharge is not possible, and that cycle life is shortened. The cathode also has problems. "The problem," Kanamura points out, "is the high resistance of the insertion and desorption reactions of Mg ions into the oxide or sulfide." In addition, the proper combination of electrolyte and separator has not been found, and, although many researchers have tried, the overall picture of Mg metal batteries remains elusive.
20% increase in capacity by adding different metals — High cycle characteristic of more than 100 cycles achieved
With this Subteam, Kanamura launched a new joint research project between researchers specializing in electrolyte development and researchers specializing in Mg alloy development as a structural material, and they are searching for an optimal anode. As a result, they were the first to discover that electrochemical activity can be greatly improved by adding an extremely small amount of a different metal, with an atomic concentration of 0.3 percent, to a Mg metal material with controlled crystal orientation and composed of crystal grains as small as 20 micrometers (one micrometer is one-millionth of a meter).
He commented, "When calcium (Ca) is added to an alloy material (MgCa) and then used for the anode, the battery's capacity is improved by about 20% when compared to using pure Mg metal as the anode." (Fig. 1)
Fig. 1: Microstructure of the Mg-Ca alloy that was developed.
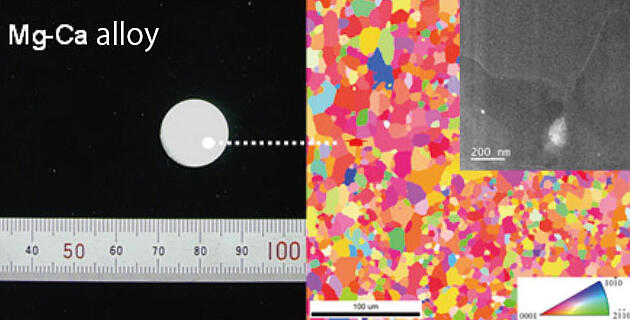
Next, the Subteam searched for cathode materials that would suppress cycle degradation. Candidates included sulfide, sulfur, and oxide materials, but, because sulfide and sulfur materials have low voltage, they focused on oxide materials. As a result, they succeeded in achieving both high capacity and high cycle characteristics with ZnMnO3, an oxide composed of zinc (Zn) and manganese (Mn) (Fig. 2). "We have achieved high cycle characteristics, stability over 100 cycles of charging and discharging for more than 100 days, while maintaining a capacity of about 100 milliampere-hours per gram at a high potential of 2 to 3 volts." Following this, Mn oxide, in which Mg ions can be more reversibly inserted and removed, has also been proposed as a new cathode active material.
Fig. 2: Schematic of the ZnMnO3 cathode material.
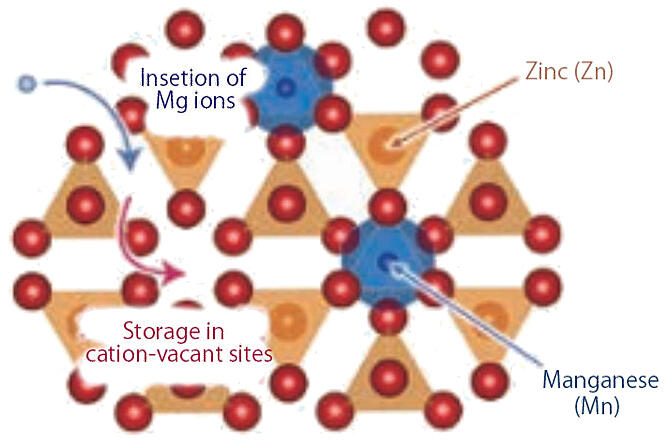
A battery's electrolyte is also essential, and it was found that an electrolyte solution using weakly coordinating anions, and a three-dimensional-ordered porous structure for the separator, are effective for an electrolyte solution. An Mg metal battery was formed by combining these two components, and full-cell operation of the battery has already been achieved.
Kanamura confidently stated, "This is the first time that anyone has understood the overall picture of Mg metal batteries." However, he has set ambitious targets for practical application, such as an energy density of 300 watt-hours per kilogram (Wh/kg) and 300 or more cycles. In the future, the Subteam will narrow down the necessary materials and components from the perspective of actual battery design and take on the challenge of full-cell verification.
To set tasks, work backwards from the goal: Working together to create the 'ultimate storage battery'
On the other hand, the Metal-Air Battery Subteam is working on storage batteries that literally use 'air.' The cathodes in these batteries use oxygen from the air and the anodes use Li (Fig. 3). Their theoretical energy density by weight is much higher than that of LIBs, and they are said to be the 'ultimate storage battery.'
Fig. 3: Schematic of a metal-air battery.
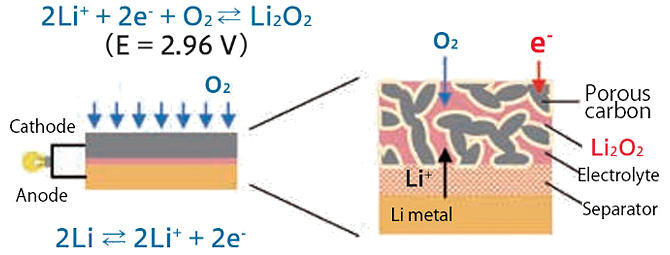
However, metal-air batteries are extremely difficult to develop. In addition to the difficulty of developing fundamental technologies such as electrodes and electrolytes, there are many cases where the fundamental technologies interfere with each other when they are integrated into a battery system, which prevents the desired performance from being achieved. Another major problem is that using oxygen as an active material generates reactive oxygen species that decompose the positive electrode and the electrolyte.
Therefore, Nakanishi, who is at the Graduate School of Engineering Science at Osaka University was appointed Subteam Leader in 2018 with the expectation that he would help to solve these problems. In order to achieve significant results in the remaining research period, he initiated a fundamental review. "We restructured the Subteam, changing from a major goal shared by the team. Instead, we now use back casting to set tasks, and then we address them together as a team. As a mechanism to achieve this, we placed the 'Centralized Research Laboratory,' which is responsible for the integrated evaluation of batteries for each fundamental technology, at the center of our Subteam" (Fig. 4).
Fig. 4: Structure of the Metal-Air Battery Subteam.
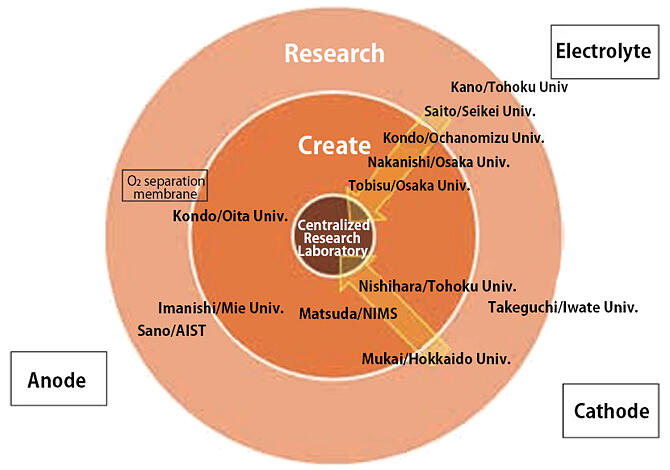
■ Propose material chemical solutions for breakthroughs
Each research group's individual results are re-evaluated by the Centralized Research Laboratory from the viewpoint of using them as actual batteries. The evaluation results are then promptly fed back to each research group. This loop has enabled efficient sharing and accumulation of results. Additionally, researchers in organic synthesis and carbon nanomaterials, who had never studied battery development, were invited to join the Subteam to lay the groundwork for scientific breakthroughs.
Breakthrough in the development of electrodes and electrolytes: Achieving 500 Wh/kg levels
One of the results of this process is a metal-air battery with an energy density at the 500 Wh/kg level. In a metal-air battery, Li+ eluted from the anode into the electrolyte combines with oxygen reduction products to deposit lithium peroxide (Li2O2) on the cathode.
Because the discharge capacity is determined by the amount of Li2O2 eluted, the cathode should be made of a material with high porosity and high specific surface area, and the electrolyte must have the function of progressing the electrode reaction in a highly reversible manner to improve cycle performance.
In discussing this breakthrough, Nakanishi said, "We have developed a new porous carbon cathode and an electrolyte containing a redox mediator that improves the reversibility of the electrode reaction." In order to further integrate these achievements into technologies that contribute to practical use, it is important to acquire solid fundamental knowledge. Based on this idea, Nakanishi has made remarkable achievements in improving the reversibility of cathode reactions. He skillfully used the oxygen isotopes 16O2 and 18O2 to analyze the Li2O2 stacking structure before and after discharge. As a result of this research, he was able to successfully identify the actual reaction field where both discharge and charge reactions happened. This finding provided a major hint for improving the cycle life of Li-O2 batteries.
The Subteam's future goal is to increase cycle life while maintaining an energy density of 500 Wh/kg. To achieve this, there are many issues to be solved, such as controlling cathode reactions, developing new electrolyte solutions, and gaining a molecular understanding of battery reactions. Smiling while speaking about future prospects in the development of air batteries, Nakanishi stated, "The road ahead will be difficult, and we will probably repeatedly fail. However, we can learn a lot from our failures and then improve. I believe that accumulating these lessons and knowledge will open up new possibilities and lead us to our next achievement."
(Article: Kazuyuki Katayanagi; Photography: Hideki Ishihara)