In the research field of life science, genetic modification technologies, starting with genome editing, are emerging one after the other. Among them, artificial chromosomes derived from humans and mice are attracting attention. When used as a genetic vehicle (vector), they have the advantage of being able to transfer large genes into various mammalian cells and individuals. Professor Yasuhiro Kazuki of the Faculty of Medicine, Tottori University School of Life Science, who serves as Deputy Director of the Chromosome Engineering Research Center at Tottori University, aims to contribute to the treatment of refractory diseases and drug discovery using unique chromosome engineering technology.
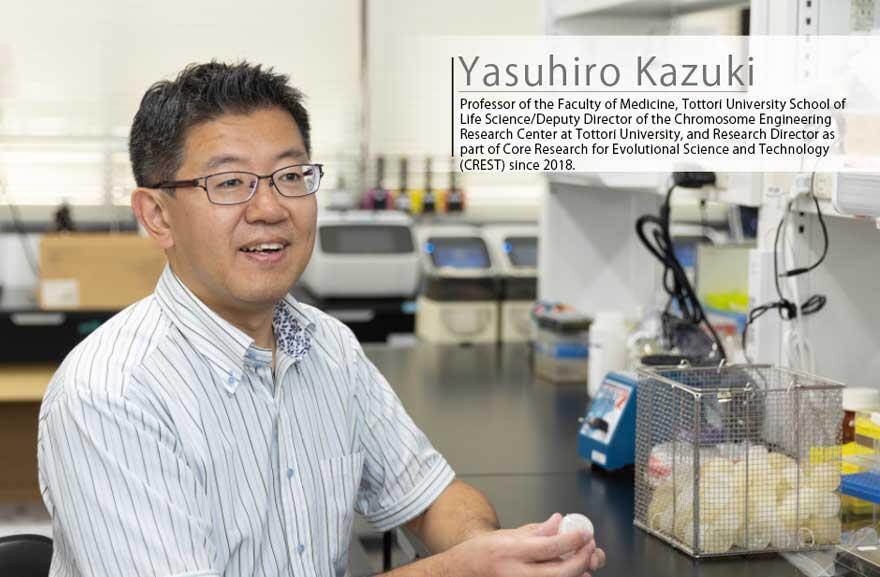
No limit to gene loading size: Pursuing this path was inspired by a research paper
Technology to transfer human genes into other mammalian cells is essential for gene therapy and clarification of disease. When transferring a gene into a cell, the vehicle that carries it is called a "vector." Conventional gene transfer vectors include viral vectors and non-viral vectors, but only small genes can be transferred. Human artificial chromosomes (HACs) solved this problem.
Humans have as many as 20,000 to 30,000 genes on 46 chromosomes. If you take one of these chromosomes and remove all the genes, leaving only the telomeres at both ends and the centromere in the center, you obtain a HAC. A target gene can be loaded onto the HAC vector, which is an empty vehicle, and transferred into various cells and animals. "Conventional gene transfer vectors can carry only 10 to 50 kilobases (base pairs), but the HAC vectors have no limit to loading size and have the advantage of being able to carry 100 times more genes than conventional vectors," said Kazuki.
He continued by saying that his decision to pursue a career in life science was inspired by an article he read in 1995 about Hokkaido University's success in Japan's first reported use of gene therapy. Interested in genetic research, he entered the Faculty of Medicine, Tottori University, School of Life Science, which aims to foster researchers in the field of medicine, as a seventh-year student. In 1997, Professor Mitsuo Oshimura of the same faculty (currently Professor Emeritus at Tottori University) published a paper in Nature Genetics in which he revealed that a single human chromosome fragment transferred into mice was functional. Stimulated by his paper on the generation of the "transchromosomic mouse," which showed for the first time in the world that externally transferred human chromosomal fragments can be transmitted to offspring, Kazuki decided to pursue a career in chromosome engineering.
Beginning research targeting muscular dystrophy: Results achieved through fusion with induced pluripotent stem (iPS) cell technology
Kazuki worked on creating a HAC vector, an empty vehicle, based on human chromosome 21. Using a similar method, he succeeded in creating a mouse artificial chromosome (MAC) vector based on mouse chromosome 11 (Figure 1). Desired genes can be freely loaded onto these artificial chromosomes. With these "designer chromosomes," basic research was launched into fields such as clarification of gene functions, production of disease model cells and animals, and research into applying these endeavors to discovery and development of drug treatments. In research that takes advantage of the characteristics of HAC vectors, Kazuki has been working on research targeting Duchenne muscular dystrophy (DMD), an intractable disease.
Figure 1:Development of human artificial chromosome (HAC)/mouse artificial chromosome (MAC) technology
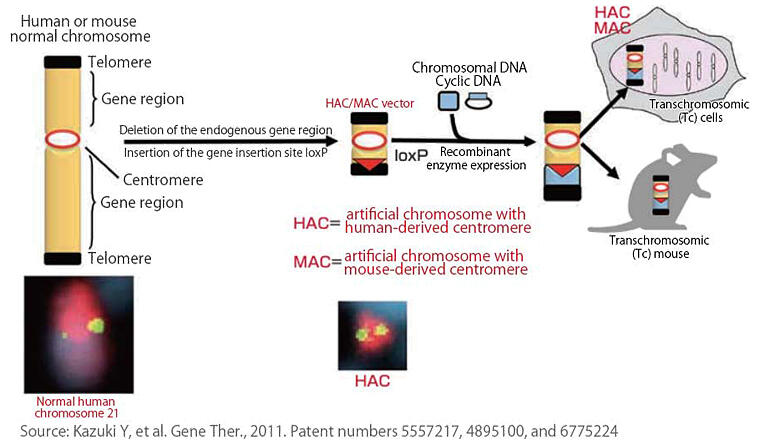
DMD is caused by a malfunctioning gene called dystrophin that triggers gradual muscle weakness and serious skeletal muscle disorders in approximately 1 in 3,500 people. The dystrophin gene (DYS) that causes the disease is one of the largest genes in humans at 2.4 megabases and cannot be delivered by conventional vectors. Kazuki wondered if it would be possible to load a normal DYS gene onto a HAC, which could then deliver a large gene as-is.
At the time the research started, Professor Shinya Yamanaka of the Center for iPS Cell Research and Application, Kyoto University (currently Professor Emeritus and Director Emeritus of the Center) had reported the successful production of iPS cells. Kazuki proceeded with research to see if combining these technologies would lead to a treatment. The HAC vector with normal DYS genes was transferred into muscle stem cells collected from a DMD mouse model. After increasing the number of stem cells and inducing their differentiation into myoblasts, they were injected and transplanted into the arteries of the mouse, resulting in improved motor function. The results of this research attracted worldwide attention as fundamental for the application of HACs to gene therapy (Figure 2).
Figure 2:Gene and cell therapy for Duchenne muscular dystrophy (DMD)
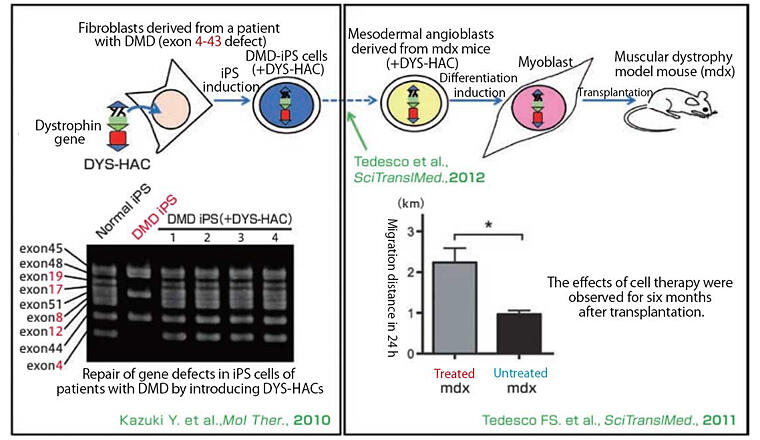
Three projects being performed concurrently — From basic technology to industrial applications
Regarding the application of HAC to gene therapy, going straight to drug development and therapeutic research in a single step is difficult. Kazuki believed that basic research is most important, and his research, under the title "Genome writing and application using human/mouse artificial chromosome," was accepted into the JST (Japan Science and Technology Agency) CREST program in 2018. Genome synthesis research (genome writing) on mammalian genomes, including human cells, had made little progress worldwide. This was because of the difficulty of gene manipulation and transfer at the chromosome level.
Since Kazuki had previously conducted research utilizing human chromosomes themselves as vectors, he decided to work on three projects concurrently at CREST focusing on HAC/MAC vectors: (1) fundamental technology development, (2) elucidation of the operative principle of genome sequence, and (3) industrial and medical application. His group is in charge of the fundamental research and is working with researchers from the Tokyo University of Pharmacy and Life Science, the Tokyo Metropolitan Institute of Medical Science, the University of Tsukuba, and the Exploratory Research Center on Life and Living Systems (ExCELLS) of the National Institute of Natural Sciences (Figure 3).
Figure 3:Organization chart of CREST
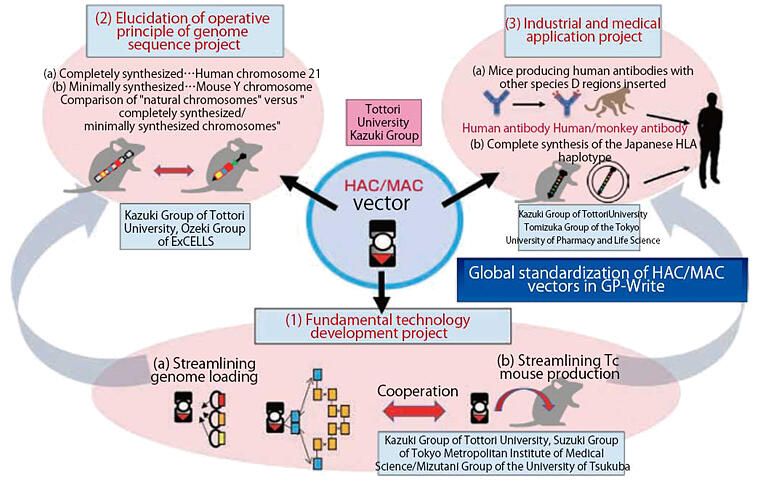
"The goal of CREST is to make artificial chromosome technology a global standard for genome writing research," says Kazuki. A major feature is that desired genetic information can be written into the genome. Conventional genome research has focused on a reading approach, and it is still fresh in our minds that the entire human genome was decoded in the 2000s. Genome writing, which adds new functions to genomes, is on the horizon and is a goal of Kazuki.
Creation of a Down Syndrome rat model and pigs with the muscular dystrophy gene
Mice and rats are used as experimental animals at the basic research stage to investigate therapeutic effects on human diseases. One of the outstanding achievements of CREST is the creation of a rat model of Down syndrome. Down syndrome is a disease caused by the trisomy of human chromosome 21. Kazuki has been researching introducing this chromosome 21 into mice since the 2000s to clarify the pathophysiology of the disease. However, the technology at that time could not efficiently produce rats with human chromosome 21. Therefore, Kazuki used his unique chromosome engineering technology to develop human chromosome 21 carrying the green fluorescent protein gene and produce rat embryonic stem (ES) cells into which one fragment of human chromosome 21 is injected. This led to the successful creation of rats that retain human chromosome 21, which could then be passed on to offspring (Figure 4).
Figure 4:Creation of the Down syndrome rat model
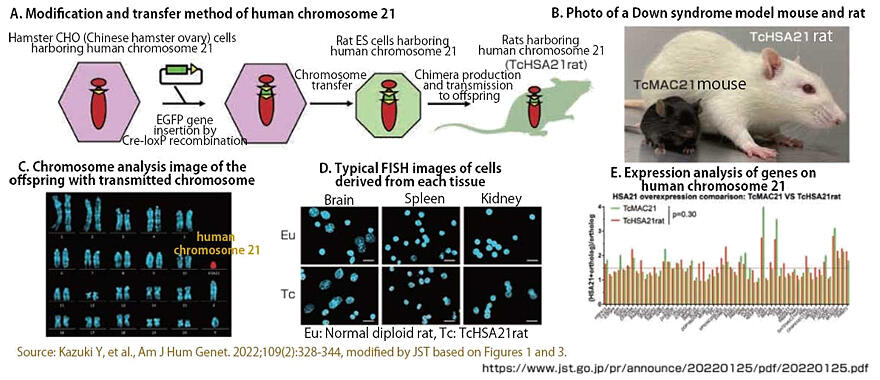
Until around 2008, genetic modification of rats was said to be technically difficult. However, this has been made possible largely owing to the 2008 report on the development of ES cells capable of generating chimeric rats that can transmit modified genes to their offspring. In Japan, Associate Professor Masumi Hirabayashi of the National Institute for Physiological Sciences of the National Institute of Natural Sciences has been conducting similar research, and joint research with Hirabayashi led to this result. The results of this research were published in Trends in Genetics, a journal in the life science field, and received a great response from overseas researchers. In addition to being an important key to elucidating the pathophysiology of Down syndrome, this research is being used for technology transfer that will lead to the development of therapeutic drugs.
Another major achievement is the transfer of human chromosomes into pigs. Pigs are suited for medical applications due to their anatomical and physiological characteristics; therefore, many genetically modified pigs have been created, but there have been technical challenges in transferring very large genes into pigs. By combining somatic cell cloning technology and artificial chromosome technology, Kazuki transferred human DYS-HACs into the fibroblasts of pigs that develop Duchenne muscular dystrophy (DMD-KO pigs). In collaborative research with Professor Hiroshi Nagashima and others at Meiji University, they succeeded in creating the world's first pigs harboring a HAC (Figure 5).
Figure 5:Creation of HAC-harboring, genetically modified pigs
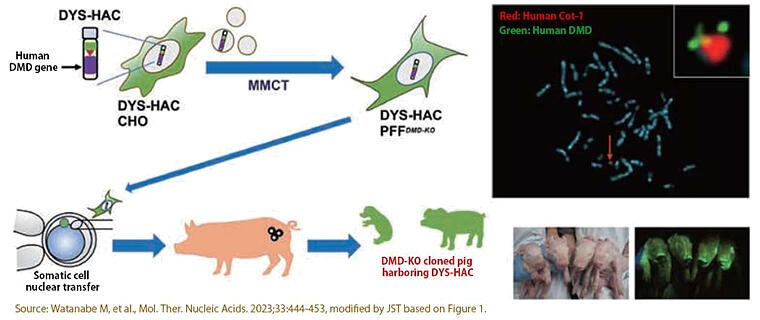
Furthermore, Kazuki has succeeded in creating "fully human antibody-producing mice" into which artificial chromosomes incorporated with full-length human antibody genes (Figure 6) are introduced. Since this mouse reproduces the antibody repertoire (diversity) seen in humans, it is expected to contribute to the development of antibody drugs that are highly effective against diseases and safe. "I would be happy if the wide distribution of fully human antibody-producing mice domestically and internationally by Tottori University could lead to the development of therapeutic drugs for cancer and emerging and re-emerging infectious diseases," says Kazuki with great enthusiasm for the future.
Figure 6:Creation of a fully human antibody-producing mouse
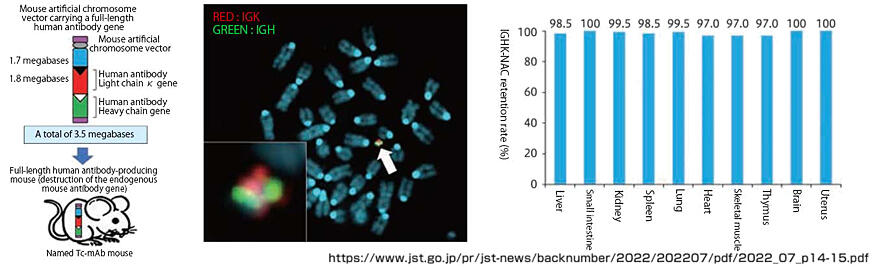
Regional industry-government-academia collaboration center launched — Seeking to create a better research environment
In October 2022, Kazuki assumed his position as professor at the Faculty of Medicine, Tottori University School of Life Science. He serves as Deputy Director of the Chromosome Engineering Research Center at Tottori University, which was established in 2009 as a joint educational and research facility between Tottori Prefecture and Tottori University, and as Director of the Tottori Drug Discovery Demonstration Center, which is managed and operated by the Chromosome Engineering Research Center. Next to the Center, the Tottori Bio Frontier, which is managed and operated by Tottori Prefecture, has been constructed as a base for the practical application of research results.
These facilities are hubs for regional industry-government-academia collaboration with chromosome engineering technology as its core and hubs for university-launched ventures in collaboration with the Chromosome Engineering Research Center. "Pharmaceutical companies and others are participating in collaborative research for which the Tottori University School of Medicine's Department of Life Sciences and the Chromosome Engineering Research Center are responsible for basic research and human resource development that will become the seeds for treatment and drug discovery, while the Tottori Bio Frontier provides early-stage support and the Tottori Drug Discovery Demonstration Center supports efforts to bring drugs to market," he says, expressing his hopes for the development of medical care and drug discovery originating from Tottori. Kazuki says that his motivation for research is to help patients suffering from diseases. He emphasizes the importance of promoting both basic and drug discovery research in tandem with vision and passion. "To achieve this, I believe it is important to create an environment where the younger generation, including myself, can freely and excitedly conduct research," he said. Currently, there are no pharmaceutical company bases or factories in Tottori Prefecture. If a university-launched venture grows, it may be possible for students to find employment after graduation, bring together medical and drug discovery personnel from within and outside the prefecture, and revitalize research. While keeping an eye on the virtuous cycle of education and research, Kazuki will continue to pursue research into the treatment of refractory diseases and drug discovery.
(TEXT: Sachiko Ito, PHOTO: Hideki Isihara)
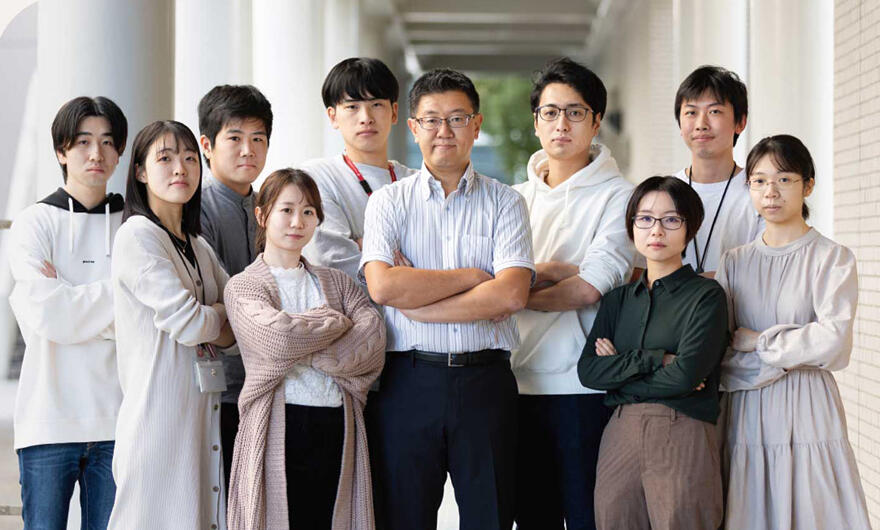