-
- Writer
- Yoshitaka Arafune
-
- Interviewer
- Yūya Nishimura
-
- Editor
- Eriko Masamura
Quantum computers are currently attracting worldwide attention. In Japan, the development of quantum computers has been selected as one of the goals of the Moonshot R&D program and is being promoted throughout the country.
Esse-sense interviewed Dr. Tsuyoshi Yamamoto of NEC Corporation—project manager of the company's quantum computer project—about his career and the challenges he faces as he looks to the future, including the transition from superconductivity research to quantum computer research, followed by research into readout technology. He is also working on the integration of qubits through the Moonshot research project. Yamamoto says that there is now a need to bring together private-sector technologies developed in different fields. This article reports on the activities undertaken at the forefront of this effort.
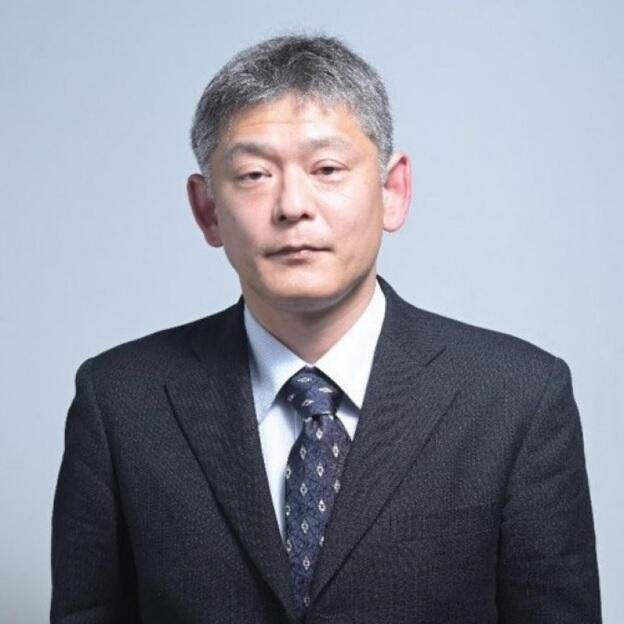
NEC Corporation Secure System Platform Research Laboratories
The impact of creating superconductors

Dr. Yamamoto, I heard that you were aiming to study chemistry right after entering the University of Tokyo. But you eventually went on to the Department of Applied Physics, in the Faculty of Engineering. Why did you change your field?

I wanted to go into chemistry when I started university, simply because I preferred chemistry to physics when I was in high school. I found organic synthesis particularly interesting and that was my starting point.
But when I actually took classes at university, I got the impression that chemistry seemed to be divided into specialized fields, whereas physics seemed to have a wider range of fields to choose from. At that time, I was not sure what I wanted to do, and I started to get interested in physics, which seemed to offer a wider range of possibilities.
Nishimura: What led you to study superconductivity in physics?
Yamamoto: When I was in my third year of my undergraduate studies, we had a class where we had to visit several laboratories, perform simple experiments related to each research topic, and write a report. I went to the high temperature superconductivity lab for that class.
The experiment we worked on involved mixing and baking several metal oxides and other powders to make a ceramic sample, cooling the sample in liquid helium the next day, and measuring its electrical resistance. Then, when I cooled the ceramic sample to a temperature of about −180℃, I witnessed the phenomenon of superconductivity, in which electrical resistance becomes zero.
Nishimura: It is called high-temperature superconductivity, but it occurs at temperatures as low as −180℃. It's a bit different from what I imagined.
Yamamoto: Historically, superconductivity was first discovered by cooling mercury to about −269℃. Since then, research has been carried out on materials in which superconductivity occurs at higher temperatures. High-temperature superconductors are a series of copper oxide superconductors first discovered in 1986, some of which exhibit superconductivity at temperatures well above the liquid nitrogen temperature of −196℃.
Returning to the story about the experiment in my third year of undergrad, I was surprised to see a strange phenomenon that would not normally occur, in the sample I, who did not know much about research, actually made. I did not know much about superconductivity at the time, but I still got the feeling from those experiments that "it just looks amazing" and "this stuff happens in reality." It made a very big impression on me.
Looking back, I might have been already interested in manufacturing even then. It was a great experience for me to realize that not only in chemistry but also in physics I can learn manufacturing, and I think it helped to develop a serious desire to study such a field.
From superconductivity to quantum computers
Nishimura: After completing your undergraduate studies, you went on to graduate school and joined NEC after completing your Ph.D. program. What made you decide to work there?
Yamamoto: I wanted to continue my research into condensed matter physics after completing my Ph.D. degree. At that time, there was a very well-known research group at NEC, and their research was very close to the research I was doing at university. So, I applied to NEC, hoping to join that group.
But the team I wanted to join didn't have any vacancies, and so I wasn't taken on. However, I was told that another team was looking for someone, so I went for another interview at NEC. I ended up joining the superconducting quantum computer team, where I am now.
Nishimura: Did you have any conflicts when you decided to work for a different team than the one you originally wanted to join?
Yamamoto: Not really. At the time, I did not know much about quantum computing, but after researching it, it seemed like a very broad field. I also felt that I could conduct research in a field that was directly related to the application of creating new computers and is close to society, so I was very eager to take on this challenge.
Of course, it was a departure from the high-temperature superconductivity research I had been working on, but it was close to my background because it deals with superconducting devices. Several of these factors, with which I felt a kinship also encouraged me to enter this field.
The dawn of quantum computer research
Nishimura: Quantum computers are now becoming better known today, but what was the situation like back then?
Yamamoto: I think that the only Japanese companies doing research on superconducting quantum computers were NEC Fundamental Research Laboratories and NTT Basic Research Laboratories. Meanwhile, at universities, several groups were conducting research from the academic perspective of mesoscopic system (*) physics, which studies physical phenomena that occur at the scale of about 100 nanometers (10 millionths of a meter) using the microfabrication technology of semiconductors.
At the same time, several groups at universities and national research institutes in the United States and Europe were working on superconducting quantum computers. At that time, I think that only a few companies were working on quantum computers, including non-superconducting ones.
- (*)Mesoscopic system: A system with an area between micro and macro scales
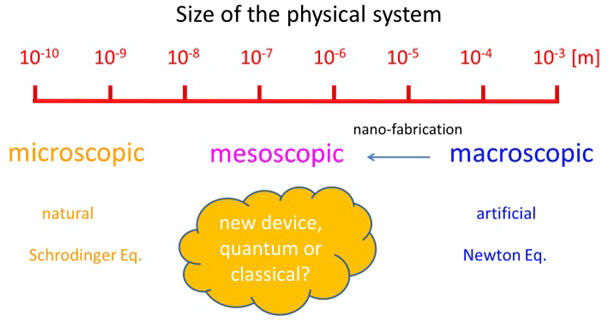
Nishimura: By the way, why did NEC start research on quantum computers?
Yamamoto: I don't know much about this because it was before I joined the company, but at NEC, Jaw-Shen Tsai (currently a professor at Tokyo University of Science) and Yasunobu Nakamura (currently a professor at the University of Tokyo) were researching a single-electron transistor that operates with a single electron.
In the process, they became interested in what happens when a single-electron transistor is made from a superconductor and pursued research in that direction as well. I think that they were motivated by curiosity to pursue their research rather than thinking specifically about quantum computers. This research had a strong academic aspect, but I think it was possible to do such research at NEC Fundamental Research Laboratories at that time.
I am told that at some point in the research on single-electron transistors made of superconductors, there was an idea of using them in the "quantum bit" of a quantum computer.
Nishimura: That is an interesting development. At first, they were making transistors with superconductors, but it turned out that they could actually be used as quantum bits—the computational elements of a quantum computer.
Yamamoto: Exactly. When I joined NEC, research on quantum bits had already started. My first work at NEC was to realize a two-qubit gate operation—the basic operation of a quantum computer—using single-electron transistor-type superconducting qubits, which were already capable of one-bit operation. In that sense, I was also working with transistor devices to create quantum bits.
The Josephson effect, which even Nobel laureates could not understand...
Nishimura: I have heard that the "Josephson junction" and the "tunneling effect" it produces are important for the superconductor quantum bit you were researching at NEC. Can you tell me what Josephson junctions and the tunneling effect are all about?
Yamamoto: A Josephson junction is a very thin layer of insulator sandwiched between two superconductors. Since insulators do not conduct electricity, it is as if the path is blocked by a high wall.
In the macroscopic world in which we normally live, if you throw a ball at a wall, it will bounce back. However, in the microscopic world of moving electrons and so on, there is a certain probability that the ball will slip through the wall and go to the other side of the wall. This is the tunneling effect.
The tunneling effect occurs not only in superconductors. A thin film of insulator sandwiched between two conductors is called a "tunnel junction," and the tunneling effect also occurs in this case. A Josephson junction replaces a conductor with a superconductor. In the case of a conductor, you can imagine electrons passing through the wall one by one, but in the case of a superconductor, a pair of electrons—or a "Cooper pair"—passes directly through the wall.
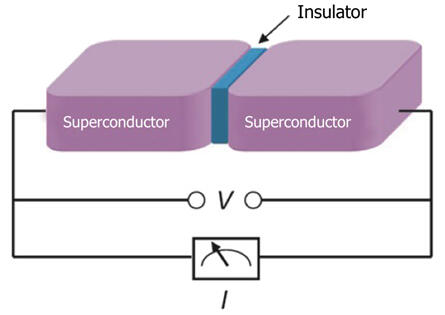
Nishimura: Can you break it down a bit more?
Yamamoto: This is a bit off-topic, but the Josephson effect is a phenomenon that was first theoretically predicted by the British theoretical physicist Josephson.
Josephson was still a graduate student when he made this prediction. That may not be the reason, but initially, there was some skepticism about his prediction, and Bardeen—who won the Nobel Prize for his theory of superconductivity—disagreed. Finally, in the year following Josephson's prediction, experiments confirmed that the theory was correct. It's a phenomenon so difficult that even Nobel laureates couldn't figure it out right away, so it's no wonder it's not immediately obvious.
Nishimura: The tunneling effect is a quantum mechanical phenomenon, so it is very microscopic in scale. Does the same thing happen on a slightly larger scale in superconductors? One can think of superconductivity as several electrons behaving together and flowing in the same direction. I was wondering if that would cause the tunneling effect to happen on a slightly larger scale than in ordinary conductors.
Yamamoto: The fact that the tunneling effect occurs on a microscopic scale remains the same, but in the case of Josephson junctions, the quantum effects appear on a macroscopic scale because the metal forming the tunnel junction is a superconductor. In the case of iron, for example, there are an uncountably large number of electrons in a lump of a few dozen grams. If we try to precisely describe the microscopic properties of metals, we need as many degrees of freedom as the number of electrons, which is very difficult to calculate.
But when superconductivity occurs, countless numbers of electrons in an object work together. In a single superconductor, no matter how many electrons there are, there will be only one degree of freedom (more precisely, one complex parameter with amplitude and phase) and one unified state.
In the case of the Josephson junction mentioned earlier, there are two superconductors across an insulator film, so there are two degrees of freedom. In other words, the current through the Josephson junction can now be described by two parameters.
Nishimura: That makes it so much easier to think about.
Yamamoto: Superconductivity, also known as a macroscopic quantum state, is a dramatic phenomenon in which quantum effects manifest themselves on a macroscopic scale. The myriads of electrons that behave individually at room temperature suddenly begin to act in concert at temperatures below a certain level. This is true regardless of the size of the superconductor.
Nishimura: So, it can be a size that we can normally touch? That's amazing.
Critical readout device in quantum computers
Nishimura: Speaking of which, did the research on quantum bits that you first worked on at NEC go well?
Yamamoto: As I mentioned a little earlier, when I first joined the company, I worked on the development of a two-qubit gate, which is the basic operation of quantum computation using two qubits. Although that research produced the expected results, there were still various technical challenges to be overcome in order to actually perform quantum computation with superconducting qubits. A very important part of this was to improve the performance of the readout, which was to read out the state of the qubits quickly and accurately. Not only within NEC, but researchers around the world were working on similar aspects of the aforementioned problem.
It was in this context—I think it was in 2004—that a new idea emerged to use microwave photons in reading out the state of qubits. The previous approach was to distinguish between 0s and 1s by the magnitude of the current flowing through the qubit. In contrast, the new method involved irradiating microwave photons on a resonator coupled to a qubit and measuring its response to determine the qubit's state.
In the quantum world, light is represented as a photon. The microwaves used in this method are very weak and are used to determine the state of a qubit with only about one photon as an average value. Therefore, a major challenge was how to accurately measure these weak microwaves.
If you make a number of measurements and average those results, you can get a reasonably accurate result, but that cannot be used for error correction. It is not practical if 0 and 1 cannot be clearly distinguished in a single measurement.
Nishimura: Why is readout a big problem?
Yamamoto: It's because it affects the computational accuracy of quantum computers. No matter how accurately a quantum computer performs calculations, it is useless if you cannot retrieve the results at the end. The result of a quantum computer calculation is obtained by reading the state of a qubit and determining whether it is 0 or 1. But if the readout results are wrong about 3 times out of 10, you are in trouble. It must be read correctly all the time.
Fault-tolerant quantum computers also need to check in real-time whether an error has occurred during the course of computation. In such cases, the readout technique is very important because the state of the qubit is read out even during the computation.
Nishimura: I see.
Yamamoto: As we continued our research on the readout technology, we realized that a high-performance amplifier is necessary to measure weak microwaves. One of the amplifiers we focused on was the "parametron".
The parametron is a computer element, invented in the 1950s by Eiichi Goto, a graduate student at the University of Tokyo, and commercially marketed by NEC in 1958 as a parametron-based digital computer. However, its use later declined as transistor-based computers became more popular.
Although the parametron itself is not an amplifier, it can be made to work as an amplifier by slightly changing the way it works. This is called a parametric amplifier. Parametric amplifiers were well-known devices before we started research on them, but around 2006 or 2007, because we thought that they could be used to read out qubits. We were able to actually build such a device, verify its operation, and publish the results in a paper in 2008.
The readout method also depends very much on the type of qubit used. Since a qubit whose state cannot be read is completely useless, the development of a qubit must be accompanied by the development of an accurate readout method. In other words, as the quantum bit has evolved, so has the readout method. Currently, the standard readout method for superconducting quantum computers is to use parametric amplifiers, but it is not clear whether this will always be the case.
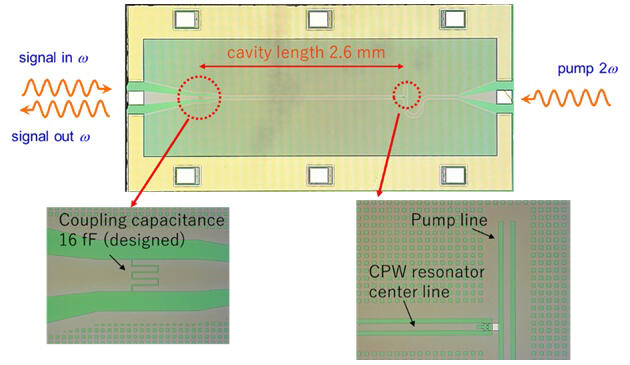
Engaging in research on quantum annealing technology
Nishimura: After that, you went on to study at the University of California, Santa Barbara. What did you do while you were there?
Yamamoto: I went to the University of California, Santa Barbara, in 2009. There was a professor there, Prof. John Martinis, who was very famous in the world of superconducting quantum-computer research, and I worked in his lab. I was experimenting with the qubits that Prof. Martinis and his colleagues have developed to realize two-qubit gate operations.
Actually, after I returned to Japan, Prof. Martinis moved his entire lab to Google. Google's quantum computer is based on the technology developed in his lab.
Nishimura: What did you do after you returned to Japan?
Yamamoto: I returned to Japan in 2010 and continued working on superconducting qubits for the next 3−4 years. During that time, we improved the parametric amplifier and developed a parametric oscillator. In fact, this is nothing more than the realization of what used to be called a parametron in a superconducting circuit. If a parametric amplifier is supplied with a large amount of energy from an external source, it acts as an oscillator rather than an amplifier. It was also found that the efficiency of reading out qubits is significantly increased when a parametric oscillator is used. So, we developed a qubit readout technology using this technology. We also applied it to the development of a single-photon detector that resolves and reads out microwave photons one by one.
Nishimura: I understand that even you, Dr. Yamamoto, who has been involved in quantum computers research since you joined NEC, have taken a step back from this field.
Yamamoto: I took a break from quantum-computer research once in 2014, when my work on parametric oscillators and other projects was completed. It was around the time that D-Wave, a Canadian company, commercialized a quantum annealing machine that runs on about 500 qubits, and quantum annealing was a particularly hot topic. It was also around this time that Prof. Martinis' lab, which I had belonged to as a visiting researcher, moved to Google.
Among Japanese companies, Hitachi and Fujitsu were working on developing dedicated hardware for non-quantum annealing to provide services for solving various optimization problems. I think they felt that the practical application of fault-tolerant quantum computers was still some time away.
Meanwhile, as far as quantum annealing was concerned, D-Wave had developed a machine with several hundred qubits. There was a lot of sensational news about how D-Wave's machine could solve certain problems 100 million times faster than ordinary computers. I think that this is the reason why Japanese companies have started to focus on quantum computers, especially quantum annealing.
Nishimura: The quantum-annealing machine is different from the quantum computer that you and your team had been working on until then, isn't it?
Yamamoto: It is. Quantum-annealing machines are specialized in solving combinatorial optimization problems such as the "traveling salesperson problem." What I have been working on since I joined NEC is a gate-based quantum computer, in which a combination of quantum gates is used to run a computational program that can perform more general computations.
Nishimura: So, Dr. Yamamoto, you have been working exclusively on gated quantum computers?
Yamamoto: That is not the case. In fact, around 2016, several domestic and overseas groups proposed that quantum annealing could be achieved using parametric oscillators as computational elements.
I have studied parametric amplifiers with great passion. When I learned that such a device could be used as a quantum computer if used in a different way, I thought "I want to try that myself." But at the time, I was researching something else at the time and was unable to get on with it immediately.
In the meantime, quantum annealing and quantum computers began to gain momentum again in the world. I thought "Here's my chance," and proposed R&D of a quantum computer using a parametric oscillator as a computational element in a proposal-competition-like event within NEC, and the company approved the development, and here we are today. But before I knew it, the story was getting bigger and bigger, and I was no longer in a situation to enjoy it casually.
Nishimura: It is amazing to see how a bottom-up proposal from a researcher is accepted, a project team is formed, and it grows. It gives me hope that such research can be done in the company.
Yamamoto: That's true. I don't know about other companies, but I think NEC has a culture where bottom-up proposals are accepted.
How to take quantum computer technology to the next level
Nishimura: Under the Moonshot R&D program sponsored by the Cabinet Office, you are conducting research on a gate-based quantum computer, right?
Yamamoto: Yes. I am the project manager for the "Development of Integration Technologies for Superconducting Quantum Circuits" which is conducted under Moonshot Goal 6: "Realization of a fault-tolerant universal quantum computer that will revolutionize economy, industry, and security by 2050."
Nishimura: Will Moonshot integrate and deploy several technologies that you have been working on, such as quantum bits and parametric oscillators? What are your goals, and what will change before and after the Moonshot program?
Yamamoto: As part of the Moonshot R&D Program, I am conducting research on a project titled "Development of Integration Technologies for Superconducting Quantum Circuits." Last year, it was announced that the Institute of Physical and Chemical Research (RIKEN) had developed a 64-qubit quantum computer. Elsewhere, IBM last year announced the development of a processor with over 1,000 qubits.
However, it is said that more than one million qubits are needed to realize a fault-tolerant quantum computer. We do not believe that it is possible to develop a computer integrating a million qubits by an extension of current technology.
As the number of qubits increases, the number of wires increases along with the size of the chip, so the refrigerator has to get bigger. There are many barriers and constraints, and it will not be possible to achieve the goal by simply extending current technology. This problem of integration applies not only to the superconducting system, but also to other platforms such as trapped ions and cold atoms. At present, I do not believe that any physical platforms offer a clear path to the realization of a fault-tolerant quantum computer.
In this project, we are conducting R&D to overcome the current situation and develop a technology to realize the integration of qubits.
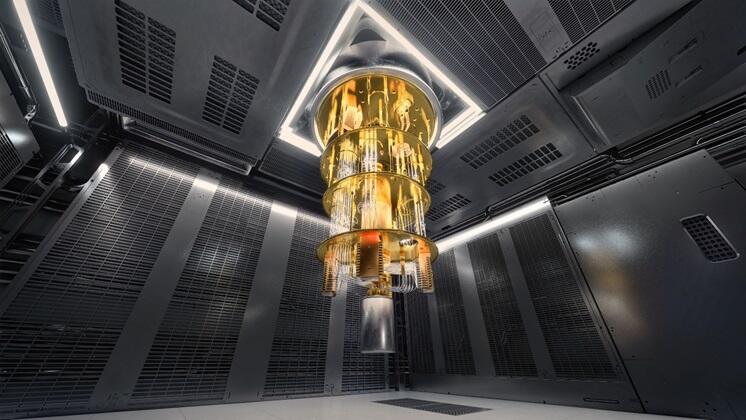
Nishimura: You mentioned that a relatively near-term goal is to ensure the operation of peripheral electronics at low temperatures. What is the purpose of this goal?
Yamamoto: The development of low-temperature electronics is necessary to reduce the number of wires used. In today's quantum computers, superconducting qubit chips are cooled to near absolute zero (−273.15℃), but their operation and readout is performed by electronics that operate at room temperature.
The room-temperature electronics and the qubits at near absolute-zero temperature are connected by coaxial cables and signals are exchanged between them, but currently one or more cables are needed for each qubit. In other words, integrating 1 million qubits with the current technology would require at least 1 million cables to be connected, making the system huge and complex.
Nishimura: Indeed.
Yamamoto: And it's not just that the machines are huge. We have to prepare room-temperature electronics for 1 million qubits. Just upping today's electronics requires large amounts of power in the megawatt range. Electronics that work at low temperatures environments are called cryoelectronics. As it develops, at least the wiring will be simpler than that of today's quantum computers.
Ideally, we would like to operate the electronics at the same temperature as the qubits. If we can run control systems at the same temperature as that of the qubits, there is no need to put the wiring on the top of the refrigerator. Of course, we will still need interfaces that allow us to enter commands and display the results of calculations. So, the number of wires connecting the cryogenic region and the room-temperature regions will not disappear entirely, but it will be dramatically reduced.
It's easy to say, but a difficult task to achieve. First, it is necessary to develop devices with very low power consumption. The cryogenic environment in which qubits are placed has a low cooling capacity. So, the temperature rises rapidly if anything that emits even a little heat is present. We need to reduce the number of wires by doing only what is absolutely necessary, by placing devices with minimal heat generation close to the qubits, and placing circuits with more processing power and a slightly higher power consumption on a stage with a slightly higher temperature, such as 4 K ( −269.15 ℃).
Nishimura: When I think of quantum computers, I think of the big chandelier-like devices you see in the news. I wondered why it is such a large device with so many cables. It was because each cable was connected to each individual qubit. For example, 64 qubits would require at least 64 cables, and in reality, it is even more.
Yamamoto: That is correct.
Nishimura: If you had a million qubits, you would need more than a million cables, which is a lot. But 1 million is only 1 mega-qubit, right? Even the old Nintendo (NES) cartridges had 2 megabits. The quantum computer that is being developed now is a computing device, and an NES cartridge is a recording medium, so there is no direct comparison, but it would be very difficult to achieve the same scale as that of the so-called electronic computers we use today.
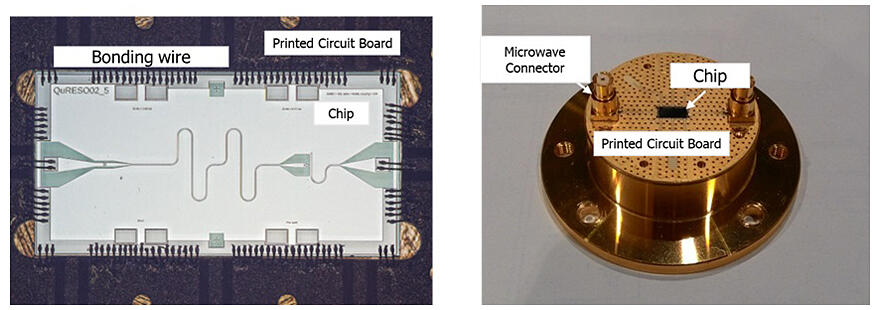
We want to bring the abilities of a lot of people together and show a roadmap
Nishimura: I understand that many people from the private sector are involved in the Moonshot project. What were your innovations in organizing the team? Are people who have not had much to do with quantum computers participating in the project?
Yamamoto: Superconducting quantum computers are developed by combining various technologies. Quantum mechanics is only a small part of what is directly involved. Of course, it is important to understand the nature and concepts of quantum mechanics, and this part of the research is not finished yet. But the importance of developments in other areas is also becoming increasingly important.
Cryoelectronics, the wiring technology I mentioned earlier, is one such example. Because it is classical electronics based on semiconductors and superconductors, knowledge of quantum mechanics and quantum computers is not always necessary. There are many things that can be handled as long as you know the interface, of what kind of signals are being exchanged. If anything, circuit design skills and knowledge of microwave engineering are more important.
In addition, there are many factors other than wiring problems that inhibit the integration of qubits. Another example is the challenge of how to manufacture qubits.
Silicon wafers used to make semiconductor integrated circuits have grown in size over the years, with 300-mm diameter wafers appearing in the 2000s. However, integrated superconducting qubits still often use wafers as small as 50 mm (2 inches) or 100 mm (4 inches) in diameter. The Josephson junctions of superconducting qubits are fabricated using electron-beam lithography, but if you try to make many qubits on a 300-mm-diameter wafer using the same method, the exposure process takes an extremely long time. There is also the problem of variations in the device parameters of Josephson junctions. Therefore, it is also necessary to develop a processing technology to fabricate uniform devices on 300-mm-diameter wafers in a short amount of time.
Thus, to promote the integration of qubits, technologies and knowledge from fields other than quantum mechanics are needed, and I believe that it is necessary to involve more and more researchers and engineers from various fields. As a result of my outreach to those with the relevant skills and expertise, we have many private-sector teams involved in our project. Many of them are also new to quantum projects.
Of course, the project is not yet complete, and there are still many areas that are missing. We need people from different fields to help us in the future.
Nishimura: So, it is important to provide a forum for open discussion across disciplines to bring together people from various fields, right?
Yamamoto: I think that is very important. We have made several such attempts. I organize workshops to introduce the initiatives and activities of the companies participating in our project, and I promote them during various speaking opportunities. These days, companies sometimes ask me, "Are you able to use our technology?"
Nishimura: So, quantum computers have a lot of problems not only with the qubit, which is at the heart of them, but also with the surrounding technology. I think that if such problems can be specifically indicated, it will be easier for various companies to get involved.
Yamamoto: I have done that to some extent, but I think it would be better to have an attitude where we keep sending out specific problems and asking, "Is there anyone who can do this?"
Nishimura: I would love to help in that area. As an immediate goal, do you aim to realize a device that integrates one million qubits?
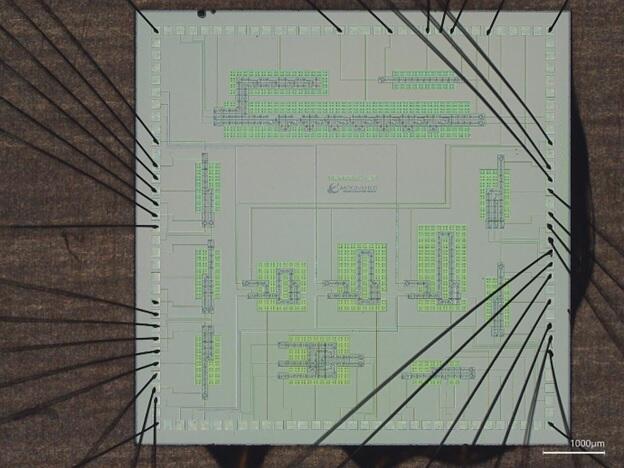
Yamamoto: I think it is difficult to integrate one million qubits all at once. The aim of the project would be to clarify the way to get there. A common challenge in all physical platforms to realize quantum computers is to draw a concrete roadmap for integrating qubits.
In the case of semiconductor integrated circuits, there is the famous "Moore's Law" proposed by Gordon Moore, one of the founders of Intel. Moore's Law states that "the number of circuit elements in an integrated circuit doubles every year." This is a rule of thumb, but in fact, integration has progressed according to this rule.
In the case of quantum computers, even if they are integrated using the current technology, they will reach their limit after about 1,000 qubits at the most. We are in a situation where we don't have a clear pathway to go beyond that, and I think that's a big problem.
In order to solve various problems and realize larger-scale systems, I think the overall design is very important, not just individual technologies. Japan has a lot of good technologies. I would like to show the way to realize a giant quantum computer with 10,000; 100,000; or 1,000,000 qubits by combining these technologies so that they fit together to form an optimal system as a quantum computer as a whole. We will conduct our research with the primary goal of providing a roadmap to guide future R&D.
Quantum computers have attracted a great deal of attention in recent years as they are expected to transform human society. As commercial and other applications begin to emerge, expectations are high. Talking to Dr. Yamamoto, it is clear that quantum computers have developed to this point thanks to the accumulation of fundamental research over a long period of time.
However, Quantum computers, are still at the basic stage. As mentioned in the interview, if you compare the number of transistors in the CPUs (central processing units) of smartphones and computers we use every day with the number of qubits in a quantum computer, you will see that the integration of qubits is a challenge for the future. Dr. Yamamoto and his colleagues are continuing their research to find means to overcome this challenge.
It has been less than 100 years since the basic technology for today's electronic computers was developed. Fifty years ago, few people could have imagined that electronic computers would be so advanced. Similarly, how far will quantum computers develop in 50 or 100 years? I am very excited to see how future research will progress.
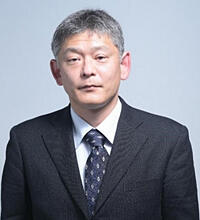
Profile
Tsuyoshi Yamamoto
NEC Corporation Secure System Platform Research Laboratories
Received a Ph.D. from the Department of Applied Physics at the Graduate School of Engineering/Faculty of Engineering, the University of Tokyo in March 2001 (D. Eng) Joined NEC Corporation in April of the same year. Since then, he has been engaged in R&D of superconducting quantum computers at the Corporation.
He was a visiting scholar at California State University, Santa Barbara, between 2009−2010. He currently holds concurrent positions at the National Institute of Advanced Industrial Science and Technology (AIST), Institute of Physical and Chemical Research (RIKEN), University of Tsukuba, and Tokyo University of Science. Since 2020, he has been the representative of the R&D project "Development of Integration Technologies for Superconducting Quantum Circuits" under Goal 6 of the Moonshot R&D project of the Cabinet Office.
This article was produced in cooperation with the JST Department of Moonshot Research and Development Program.
https://www.jst.go.jp/moonshot/en/index.html
Original article provided by esse-sense and translated by Science Japan.